Exosomes Derived from Immune Cells: The New Role of Tumor Immune Microenvironment and Tumor Therapy
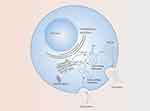
Introduction
Exosomes are 40–100nm sized vesicles released into the microenvironment by different types of cells. Exosomes contain proteins, RNA (including miRNA, LncRNA and mRNA) and lipids. They are an important medium for cell–cell interaction in the tumor microenvironment.1 Exosomes can be secreted by a variety of cells, and exosomes from tumor cells can act on other stromal cells in the tumor microenvironment, for example, they can act on vascular endothelial cells to promote angiogenesis to provide nutrition for tumors, act on other tumor cells to promote their value-added migration ability, or act on immune cells to create an immunosuppressive microenvironment, resulting in immune escape.2–4 The process of exosome production in various cells is similar, with cells producing small vesicles through endocytosis, which fuse to form early endosomes and gradually become late endosomes. With the entry of some “Cargo” such as cytosolic RNA, proteins, and lipids, late endosomes produce many intraluminal vesicles (ILVs) and evolve into multivesicular bodies (MVB); subsequently, these vesicles are released outside the cell to form exosomes.1 Under different physiological and pathological conditions, their contents are often different, reflecting the physiological and pathological states of the original host cells, which makes exosomes as reliable carriers for precision medicine and liquid biopsy.5
For most solid tumors, the traditional treatment methods include surgery, radiotherapy, chemotherapy and so on. Immunotherapy is a new treatment method in recent years. Biological immunotherapy includes several types: 1. Biological cells: such as dendritic cells (DCs) and T cells, which are immune cells in the human immune system. By enhancing the role of immune cells, they can fight against tumors;6,7 2. Immunotherapeutic drugs: for example, immune checkpoint blocking (ICB) therapy mainly prevents immune escape by targeting PD-L1, PD-1, CTLA-4, so that immune cells, especially T cells, can play an immune killing role again.8 Nowadays, more and more studies have found that immune cells also produce exosomes, which also play a double-edged sword role in the tumor microenvironment. It is worth noting that exosomes from T cells or NK cells produce immune killing effect on tumor cells, and exosomes have the ability to cross physical barriers, inherent targeting characteristics and their increased biocompatibility. It has great potential as a new drug delivery system, which may provide new possibilities for tumor immunotherapy. In this review, we summarized the roles and mechanisms of various immune cell-derived exosomes in the tumor microenvironment, and discussed the possible therapeutic effects of engineered immune cell-derived exosomes on tumors.
The Physiology of Exosomes
The Formation and Release of Immune Cell Derived Exosomes
The biogenesis of exosomes involves double invagination and subsequent plasma membrane fusion. First, the cell membrane buds inwards to form early endosome (EE), and then chimes with Golgi complex membrane to form late endosome (LE). LE selectively wraps some specific soluble components in cytoplasm, including nucleic acid, protein and lipid, and then forms intraluminal vesicle (ILV, precursor of exosomes). The ILV fuses with each other to form multiple intraluminal vesicles (MVB), which secrete to the back of the cell and outside the cell as the exosome.1,9 MVB maturation occurs through at least two mechanisms, one involving endosomal classification complex (ESCRT) – 0, – I, – II and – III required for transportation and ESCRT-related proteins (such as VPS4 and ALIX); In addition, there are also ESCRT independent regulatory mechanisms such as heteroribonucleoprotein-dependent pathway and neutral sphingomyelinase 2 dependent pathway.10–12 Recently, Wei D et al identified a new ESCRT independent mechanism regulated by RAB31, which improved our understanding of exosome formation.13 Once MVBs are produced, they can fuse with lysosomes or autophagic lysosomes, leading to the degradation of MVBs, or they can fuse with the plasma membrane and release cells as exosomes1,14 (Figure 1).
![]() |
Figure 1 The formation and release of immune cell derived exosomes and its contents. |
The Cargo of Exosomes
In recent years, with the development of gene chip technology, proteomics research and high-throughput sequencing technology, a variety of exosome contents from different cells have been screened, including 9769 proteins, 1116 lipids, 3408 mRNA and 2838 miRNA included in the Exocarta database.15 The vast majority of these contents have been confirmed to be involved in the occurrence and development of many diseases, including tumors. For example, the exosomes rich in miR-1910-3p promote the proliferation and migration of breast cancer cells,16 and the colorectal cancer exosomes miR-25-3p promote vascular permeability and angiogenesis by transferring the expression of VEGFR2, ZO-1, occludin and Claudin 5 into endothelial cells,17 Moreover, circTRPS1 derived from bladder-cancer-derived exosomes can regulate intracellular ROS balance and CD8+T cell depletion through circTRPS1/miR141-3p/GLS1 axis.18 In addition, exosomal PD-L1 have been widely discussed in recent years. Metastatic melanoma releases high-level exosomes and carries PD-L1 on its surface, thereby inhibiting the function of CD8+T cells and promoting tumor growth.19 To sum up, in-depth excavation of the contents of the exosome can not only provide reliable molecular markers for the diagnosis of various diseases, but also help to deeply understand the pathogenesis of diseases and provide a new direction for disease prevention and treatment.
The Role of Exosomes from Different Immune Cell Sources in Tumor Microenvironment
In recent years, due to the shortcomings of conventional cancer chemotherapy drugs, such as low bioavailability (the speed and extent of drug absorption into the human circulation), low improvement indicators of treatment effect, and unclear side effects, the development of drug delivery systems has gradually become a research hotspot. In principle, the drug delivery system can enhance the efficacy of anti-cancer drugs. At the same time, the increased delivery efficiency of targeted drugs also helps reduce the toxicity of drugs in the whole body. The drug delivery system has achieved better drug treatment effect in humans or animals by combining drugs and assistive compounds.20,21 Recent studies have shown that exosomes have the ability to overcome physical barriers, inherent targeting properties and their increased biocompatibility, which makes them have great potential as a new drug delivery system.22,23 In addition, exosomes from different cells (immune cells, tumor cells, stem cells, etc.) will play a role in anti-tumor therapy by different mechanisms.24,25 Jiang et al designed an exosome derived from bone marrow mesenchymal stem cells (BM-MSCs) as a dual drug delivery biological system to enhance the effect of immunogenic cell death (ICD), promote DC maturation, and reverse immunosuppression (inhibiting macrophage polarization, cytotoxic T-lymphocyte recruitment, and down regulation of Tregs).26 The engineered exosomes (HELA Exos) composed of TLR3 agonist and ICD inducer were constructed from the exosomes derived from human breast cancer cells, which can activate dendritic cells in situ and specifically induce ICD in breast cancer cells.27 Even though exosomes have potential advantages in drug delivery, the efficiency of drug loading is not high. In order to improve the loading efficiency of therapeutic drugs in exosomes, people have studied various methods, especially with the progress of micro nano processing technology, and studied the application of microfluidic technology in cell drug loading and drug delivery.28–30 Youngjin Lee et al developed a microfluidic device (Exo-Load) to detect whether DOX and PTX can be loaded into the exosomes and whether drugs can be loaded into the exosomes efficiently. In addition, saponins have been widely used as penetrants to change the properties of cell membranes. Therefore, they used saponins and DOX to increase the combined effect of permeability and shear stress induced stimulation in the microchannel of the externally loaded microfluidic device, so as to improve the efficiency of its loading into the exosome.31 Equally important is how the exosome obtains energy to establish intercellular communication. Recently, some studies have shown that there are mitochondria, adenosine triphosphate and glycolytic enzymes, which can be used as potential energy sources of exosomes.32 This chapter will elaborate on the therapeutic effect of engineered exosomes from different immune cells in tumors.
T Cells
T cells are multipotential stem cells derived from bone marrow, which are composed of a group of heterogeneous lymphocytes with different functions.33–35 In the embryonic and primary stage of human body, a part of multipotential stem cells or pre-T cells in bone marrow migrate to the thymus, differentiate and mature under the induction of thymic hormone, becoming T cells with immune activity.33–35 Then it circulates with the blood to the surrounding lymphoid organs to settle and reproduce in the respective established areas. Activated by antigen, it can differentiate and proliferate, produce effector cells and exercise its immune function.33–35
CD8+T Cells
CD8+T cells are a subgroup of T cells, also known as cytotoxic T cells, which are one of the most toxic T cells. They can kill the target cells expressing antigens; it is an important effector in anti-virus infection, acute allograft rejection and killing of tumor cells. In the tumor microenvironment, CD8+ T cells play a key role in the anti-tumor effect by releasing perforin, granzyme, TNF-α and Fas/FasL pathway.36,37 In addition, CD8+T cells can also play a role in tumor immunity by releasing cytotoxic exosomes. Tumor stroma contains a large number of mesenchymal stem cells (MSCs) and cancer-related fibroblasts (CAFs), which can promote the invasion and metastasis of tumor cells.38,39 However, the exosomes released by CD8+T cells (CD8+T-Exos) can consume mesenchymal tumor stromal cells (including MSC and CAF) and inhibit the characteristics of tumor progression such as invasion and metastasis.40 In uterine corpus endometrial cancer (UCEC), low-level miR-765 can be closely related to high proliferation, invasion and metastasis of tumor cells and poor prognosis by activating PLP2-Notch signal. In addition, as a risk factor for UCEC, estrogen/ER β can regulate miR-765/PLP2 axis and further promote tumor progression. However, CD8+T-Exos release high levels of miR-765 and limit the tumor promoting effect of estrogen on UCEC by regulating the miR-765/PLP2 axis.41 In addition to inhibiting tumor progression, Cao et al also found that FasL+ exosomes secreted by CD8+T cells activated tumor cells ERK and NF-κB pathway, and then increased the expression of MMP9, which promoted the invasion and metastasis of tumor cells.42 Interestingly, a subtype of CD8+T cells, exhausted CD8+ T cells can secrete a large number of exosomes, which can be ingested by normal CD8+ T cells, reducing their proliferative capacity (Ki67), cell activity (CD69) and IFN- γ and the production of cytokines such as IL-2, which leads to the weakening of tumor killing capacity43 (Table 1, Figure 2).
![]() |
Table 1 Role of T Cell Derived Exosomes in Tumor Microenvironment |
![]() |
Figure 2 Effects of exosomes from different types of T cells on tumor cells and other stromal cells in the tumor microenvironment. |
γδ T Cells
T cells can be divided into αβ T cells (such as CD4, CD8) and γδ T cells according to TCR. The majority of human peripheral blood lymphocyte are αβ T cells, and γδ T cells account for only 1% −5% of the total population. Although their number is very small, their strength cannot be underestimated. γδ T cells can directly kill tumor cells through apoptosis-inducing protein ligand pathway Fas-FasL, ADCC effect and its secreted cytokines (IFN-γ, TNF-α). Besides, γδ T cells can also activate B cells, DC cells, αβ T cells, NK cells in various ways (eg, act as antigen presenting cells for activation αβ T cells, or NK mediated anti-tumor cytotoxicity induced by costimulatory pathway), so as to achieve indirect killing of tumor.44,45 Recent studies have shown that γδ T cells can also increase tumor killing effect by releasing exosomes. In EBV associated tumors, γδ T cell derived exosomes (γδ T-Exos) contain death inducing ligands (FasL and TRAIL), NK cell activating receptors (NKG2D), immunostimulatory ligands (CD80 and CD86) and antigen presenting molecules (MHC class I and II).46 γδ T-Exos have a dual killing function against tumor cells and thus control EBV-associated tumors more effectively than exosomes derived from NK cells or DC cells. γδ T-Exos kills EBV-associated tumor cells through the FASL and TRAIL pathways while promoting EBV antigen-specific CD4+ and CD8+ T cell expansion to increase anti-tumor immunity.46 Similarly, in nasopharyngeal carcinoma (NPC), γδ T-Exos kills tumor cells through Fas/FasL and death receptor 5 (DR5)/TRAIL pathways. In addition, γδ T-Exos can also promote the migration of T cells to NPC cells by up regulating the expression of CCR5 ligand on NPC cells.47 Meanwhile, γδ T-Exos has synergistic effect with radiotherapy, and their combined application has great potential in the treatment of nasopharyngeal carcinoma.47 In addition, Li et al explored the role of γδ T-Exos in tumor therapy as a drug delivery system. They overexpressed miR-138 in γδ T cells with lentiviruses and subsequently isolated exosomes that both retained the cytotoxic effects of γδ T cells and transported miR-138 to oral squamous-cell carcinoma cells (OSCC). MiR-138-enriched γδ T-Exos can not only directly inhibit tumor cell proliferation, but also promote CD8+ T cell proliferation, IFN-γ secretion and cytotoxicity increase to further inhibit tumor.48 This provides the possibility of γδ-T-Exos as a drug delivery system (Table 1, Figure 2).
CD4+T Cells
CD4+T cells, also known as helper T cells, can assist CD8+ T cells to participate in cellular immune killing, clear tumor cells or virus-infected cells, and assist B cells to participate in humoral immunity. CD4+T cells can differentiate into four types of cells with different functions under the action of different cytokines and environments: Th1, Th2, regulatory T cells (Treg), Th17 cells.49,50 Interestingly, CD4+T-cell-derived exosomes (CD4+T-Exos) play different roles. Zhang et al proved through in vitro experiments and animal experiments that the CD4+T-Exos can inhibit CD8+ CTL response and anti-tumor immunity, which is unfavorable for tumor treatment, but this exosome can be used as a new immunosuppressant for the treatment of autoimmune diseases and transplantation rejection.51 In addition, CD4+T-Exos can also participate in B cell responses. The treatment of CD4+T-Exos can enhance the humoral immune response of mice inoculated with hepatitis B surface antigen (HBsAg). Compared with control mice, the level of hepatitis B surface antibody (HBsAb) in the serum of CD4+T-Exos treated mice was higher, which was antigen dependent. CD4+T-Exos can promote B cell activation, proliferation and antibody production in vitro. Using mouse T-cell lymphoma EL-4 cells, it was found that CD40L played an important role in exosome mediated B cell response52 (Table 1, Figure 2).
Tregs
Regulatory T cells are mainly divided into natural regulatory T cells (nTreg, CD4+CD25+Treg), adaptive regulatory T cells (aTreg or iTreg), CD+8 regulatory T cells (CD8+CD25+Treg) and natural killer T cells (NKT). Through direct contact, they can inhibit the activation of target cells, or secrete cytokines such as interleukin-10, inhibit immune response, and play an important role in autoimmune diseases, infectious diseases, organ transplantation, tumors and other diseases.53,54 Xie et al extracted exosomes from in vitro-expanded CD8+CD25+Treg cell culture supernatants and then evaluated their effects on CTL response and anti-tumor immunity in mice. They found that they can suppress CD8+ T cell responses and anti-tumor immunity, which may make them an option for immunotherapy of autoimmune diseases.55 CD4+CD25+Treg derived exosomes can package specific miRNA and iNOS, deliver them to T cells, disrupt cell cycle process, induce cell apoptosis, inhibit T cell proliferation, and transform immature T cells into Treg cells. These effects of exosomes play an immunosuppressive role together.56 Similarly, CD4+CD25+Treg derived exosomes can highly express CD73, which in turn plays an immunosuppressive role in immune regulation by producing adenosine.57 In addition, SIM L. Tung et al also found that miR-150-5p and miR-142-3p can be transferred from Treg to DC through exosomes derived from CD4+CD25+Treg. DC uptake of these exosomes produced a tolerance phenotype, and IL-10 increased and IL-6 production decreased after LPS stimulation.58 These studies have proved that exosomes from Treg cells play an important role in shaping the immunosuppressive microenvironment, which may help to improve the efficacy in patients receiving transplantation or suffering from autoimmune diseases (Table 1, Figure 2).
Car-T
Chimeric antigen receptor T-cell immunotherapy (CAR-T) is a type of immunotherapy in which the antigen-binding site of an antibody that recognizes a specific tumor antigen is coupled to the intracellular portion of the CD3-δ chain or FCεrIγ to form a chimeric protein in vitro, the patient’s T cells are transfected with genes that make them express chimeric antigen receptor (CAR). The patient’s T cells were “Reprogrammed” to produce large numbers of tumor-specific CAR-T cells.59,60 Shi et al found that exosomes released by CAR-T cells express CARs on their surfaces. CAR-containing exosomes have high levels of cytotoxic molecules and are used in cancer therapy. Analysis of in vitro and preclinical in vivo models suggested that CAR exosomes do not express PD-1, and that recombinant PD-L1 treatment did not impair the antitumor effects of CAR exosomes. In a preclinical in vivo model of cytokine release syndrome, administration of CAR exosomes is relatively safer compared to CAR-T therapy.61 Therefore, these data confirm that exosomes can be used as biomimetic nanovesicles, which may be a new effective strategy for the treatment of cancer. Mesothelin, also known as MSLN, is a recent hot target in CAR-T and CAR-NK therapies. Normally, MSLN is expressed in mesothelial cells, its function is associated with cell adhesion, and MSLN is overexpressed in a variety of human tumors.62 The researchers constructed anti-MSLN CAR-T cells and isolated exosomes from this cell that also retained most of the characteristics of the parental T cells.63 The results of in vitro experiments showed that anti-MSLN CAR-T cell exosomes carrying perforin and granzyme B directly killed triple-negative breast cancer cells. At the same time, this tumor-suppressive effect was also validated in animals, and high levels of CAR-T cell exosomes were found to still have lower toxicity in vivo.63 These studies make it possible for CAR-T cell-derived exosomes to become one of the immunotherapeutic schemes for cancer (Table 1, Figure 2).
Exosomes Released by T Cells Under Immunotherapy
Programmed Cell Death 1(PD-1), one of the major immune checkpoints, is expressed on a variety of immune cells, in particular, the combination of PD-1 on the surface of T cells and PD-L1 on the surface of tumor cells can inhibit the killing ability of T cells.64 Studies have shown that activated T cells can release exosomes that express PD-1 on the surface, which can competitively bind to PD-L1 on the surface of tumor cells and reduce the binding of PD-1 to PD-L1 on the surface of T cells, and then reduced T cell dysfunction.65 In recent years, anti-PD-1-based immune checkpoint therapy plays an important role in the treatment of cancer, but because of the existence of drug resistance, the combination of immune checkpoint therapy and chemoradiotherapy usually results in long-term therapeutic benefit.66 Interestingly, T cells promoted the release of exosomal miR-4315 under anti-PD1 treatment. And exosomal miR-4315 can mediate the downregulation of Bim, a pro-apoptotic protein, in tumor cells, which leads to increased resistance to conventional chemotherapy drugs.67 The researchers also found that ABT-263, a small molecule Bcl-2 inhibitor that induces apoptosis, can eliminate anti-PD1/exosomal miR-4315-induced chemotherapy resistance, which provides a new option for tumor therapy.67
Macrophages
Macrophages (abbreviated as m ø) are immune cells widely distributed in the blood and tissues of the whole body. Macrophages can phagocytize and kill intracellular parasites, bacteria, tumor cells, aging and abnormal cells, and play an important role in immune defense and immune surveillance. Macrophage is a kind of phagocyte, belonging to monocyte system. Macrophages are remarkably plastic cells that can switch from one phenotype to another. Macrophage polarization is a process in which macrophages exhibit specific phenotypes and respond functionally to microenvironment stimuli and signals encountered in each specific tissue. It has two major macrophage subsets with different functions include classically activated or inflammatory (M1) and alternatively activated or anti-inflammatory (M2) macrophages.68,69
M1 Macrophage
M1 macrophages are mainly composed of IFN-γ, granulocyte-macrophage colony stimulating factor (GM-CSF) or lipopolysaccharide (LPS); it can release a variety of pro-inflammatory factors, immune activating factors and chemokines, and play an anti-tumor role through acute pro-inflammatory reaction, immune activation reaction and cell phagocytosis.70,71 It should be noted that M1 macrophage derived exosomes (M1-Exos) can also inhibit tumor progression by directly promoting tumor cell apoptosis or enhancing tumor immune response. Serine/threonine kinase 16 (STK16) is highly expressed in lung adenocarcinoma, which can promote the proliferation of tumor cells and is closely related to the poor prognosis of lung adenocarcinoma.72 M1-Exos can carry miR-181a-5p to target ETS1 to inhibit the expression of STK16 in tumor cells, thereby reducing cell viability and promoting apoptosis of lung adenocarcinoma cells.73 In addition, M1-Exos can also carry miR-16-5p to reduce the expression of PD-L1 in gastric cancer cells, thus reducing the inhibitory effect of immune checkpoints on T cells and promoting the activation and killing ability of T cells.74 Chiara cianciaruso et al collected EVs released from TAM (TAM-EV) of mice and performed quantitative, proteomic, and lipidomic analyses of them. They found that TAM-EV presented a molecular spectrum related to Th1/M1 polarization, enhanced inflammation and immune response, and more favorable patient prognosis. TAM-EV can also promote T cell proliferation and activation.75 Interestingly, Huang et al found that M1-Exos can also play a dual role in inhibiting tumors, the M1-Exos highly express lncRNA HOTTIP, which can competitively bind miR-19a-3p and miR-19b-3p to up regulate TLR5/NF-κB signal pathway, thereby inhibiting the proliferation, invasion and metastasis of head and neck squamous cell carcinoma cells.76 In addition, it can also polarize circulating monocytes into anti-tumor M1 macrophages and increase the tumor killing capacity.76 The M1-Exos is a topic worthy of investigation, and we can continue to explore whether it could play a greater role as a regulator of other immune cell functions in the tumor microenvironment (Table 2, Figure 3).
![]() |
Table 2 Role of Macrophage-Derived Exosomes in Tumor Microenvironment |
![]() |
Figure 3 Effects of M1 and M2 macrophage derived exosomes on tumor cells and other stromal cells in the tumor microenvironment. |
M2 Macrophage
M2 macrophages are closely related to tumor microenvironment, including cytokines IL-4, IL-10 and transforming growth factor-β (TGF-β), Vitamin D3, M-CSF and prostaglandin E2 (PGE2) can induce the activation of macrophages to form M2 macrophages. It can inhibit the proliferation and activation of T cells, regulate and promote Th2 immune response, promote the growth of tumor cells, participate in tumor angiogenesis and promote tumor invasion and metastasis by secreting immunosuppressive factors, cytokines and growth factors.77,78 The role of M2 macrophage derived exosomes (M2-Exos) in tumor microenvironment is similar to that of M2 macrophages. First, M2-Exos can promote the proliferation of tumor cells. Cyclin-dependent kinase inhibitor 1B (CDKN1B) is considered to act as an inhibitor of cell cycle progression during G1/S transition. Low levels of CDKN1B are associated with tumor cell proliferation and poor prognosis.79 M2-Exos miR-221-3p promotes tumor cell proliferation and G1/S transformation by reducing the level of CDKN1B in tumor cells.80 Secondly, the tumor promoting effect of M2-Exos is reflected in enhancing the invasion and metastasis of tumor cells. M2-Exos transfer miR-501-3p to PDAC cells, thereby down regulating the expression of TGFBR3, and finally through TGF-β signal pathway promotes invasion and migration of PDAC cells.81 Similarly, in esophageal cancer, M2-Exos down regulate miR-26a and up regulate ATF2 by transferring lncRNA AFAP1-AS1 to esophageal cancer cells, an activating transcription factor that is related to poor tumor prognosis, thus promoting the invasion and metastasis of esophageal cancer.82 In medulloblastoma, M2-Exos miR-155-3p also plays a role in the invasion and migration of tumor cells.83 Tumor angiogenesis is a marker of solid tumor growth, invasion and metastasis. More and more studies have shown that macrophages are closely related to angiogenesis.84,85 M2-Exos also have such effects, for example, a study has shown that they can carry miR-155-5p and miR-221-5p into endothelial cells, and promote angiogenesis in pancreatic ductal adenocarcinoma by targeting E2F2.86
M2-Exos can also affect immune cells in the tumor microenvironment to promote tumor cell immune escape and accelerate tumor progression. In colorectal cancer, M2-Exos miR-155-5p reduces IL-6 secretion by down regulating Zinc-finger-type-containing 12B (ZC3H12B) expression in tumor cells, which leads to decreased proliferation of CD3+T cells and IFN-γ+T cells, which promoted the immune escape of tumor cells.87 Similarly, in hepatocellular carcinoma, M2-Exos miR-21-5p passes through YOD1/YAP/β-catenin pathway promotes the increase of CD8+T cell depletion subtype (PD1hi TIM3+) and reduces the killing ability of CD8+T cells.88 Interestingly, M2-Exos miR-29a-3p was able to down regulate FOXO3-AKT/GSK3β axis to promote the expression of PD-L1 on the surface of tumor cells, and finally promote the combination of PD-L1 and PD-1 on the surface of CD8+T cells to inhibit their killing function and lead to immune escape.89 In addition to affecting CD8+T cells, it also regulates the proportion of Tregs and Th17 cells, two subpopulations of CD4+T cells. Tregs can inhibit tumor immunity, while Th17 cells can regulate the activation of cytotoxic CD8+T cells. M2-Exos miR-29a-3p and miR-21-5p are transferred to CD4+T cells. They can directly inhibit STAT3 and induce the imbalance of Treg/Th17 cell ratio, producing an immunosuppressive microenvironment that promotes the progression and metastasis of epithelial ovarian cancer.90 One of the main characteristics of PDAC is its resistance to gemcitabine. Ziv Gil et al revealed that M2-Exos miR-365 impairs the activation of gemcitabine by up regulating the triphosphate nucleotide pool in cancer cells and inducing cytidine deaminase, thereby reducing the sensitivity of tumor cells to gemcitabine.91 Radiotherapy plays an important role in tumor treatment. Recent studies have found that M2-Exos LncRNA AGAP2-AS1 reduces the sensitivity of lung cancer to radiotherapy by reducing the expression of miR-296 and increasing the expression of Notch2. This seems to provide a new scheme to improve the efficacy of radiotherapy.92 In conclusion, M2-Exos in the tumor microenvironment almost preserve the same functions as macrophages, including promoting tumor cell proliferation, invasion, metastasis and angiogenesis. In addition, it can also promote immune escape by inhibiting the function of CD4+T cells or CD8+T cells (Table 2, Figure 3).
NK Cells
NK cell is a kind of innate immune cell, which is the third kind of lymphocyte in parallel with T cell and B cell. NK cells are an inborn “killer”, which can react quickly without antigen pre stimulation and produce nonspecific killing effect.93,94 As the first line of defense for human body to eliminate tumor cells, the functions of NK cells mainly include: (1) secreting cytotoxic particles such as perforin and granzyme to induce apoptosis of target cells. (2) Expression of TNF superfamily members in combination with corresponding receptors induces apoptosis of target cells, such as fas binding Fas ligand (FasL) (3) secreting a large number of cytokines (IFN-γ), growth factors and chemokines, and recruit macrophages, DC cells, T cells and other immune cells to act together. (4) It expresses Fc receptor (CD16) and mediates ADCC effect.95,96 In 2017, Ambrose y. Jong et al proposed for the first time a new, simpler and different extraction method to separate NK cell-derived exosomes (NK-Exos), and they found that NK-Exos contained cytotoxic proteins perforin (PFN), Granzyme (Gzm-A and Gzm-B) and granolysin (GNLY). At the same time, after co-culturing exosomes with tumor cells, they found that exosomes could activate caspase-3, −7 and −9 to induce tumor cell apoptosis.97 This provides a theoretical basis for the later experimental and clinical study of NK-Exos.
Byeong-Cheol Ahn et al confirmed that Fas expressed on the surface of NK-Exos combined with tumor cell FasL to induce apoptosis by activating caspase-8, caspase-3 and PARP 30. At the same time, they also discovered for the first time that NK-Exos contain TNF-α, and confirm TNF-α can affect the proliferation, survival and apoptosis of melanoma cells.98 In addition, LFA-1 and DNAM-1 can be expressed on the surface of NK-Exos, and the combination of DNAM-1 with corresponding receptors on the surface of tumor cells can also activate caspase-dependent apoptosis.99 In addition, Ambrose Y. Jong supplemented the cytotoxicity mechanism of NK-Exos. In addition to the caspase-dependent apoptosis pathway in the above studies, there are also caspase-independent pathways, including: 1. GzmA in exosomes induced degradation of SET and HMG2 proteins in tumor cells; 2. induce tumor cell mitochondria to release cytochrome C into the cytoplasm, which is an important substance in the caspase-induced apoptosis pathway; 3. GzmB and GNLY in exosomes induce apoptosis of tumor cells by inducing endoplasmic reticulum stress.100 In addition, NK-Exos can also carry tumor suppressor miR-186 to MYCN amplified neuroblastoma cells to inhibit the proliferation and migration of tumor cells.101 Interestingly, hypoxia is one of the characteristics of most solid tumors and can promote tumor progression. However, a recent study showed that hypoxia can promote the secretion, of NK-Exos and increase the expression of cytotoxic related molecules, promote tumor cell apoptosis and inhibit tumor cell migration.102 In conclusion, it can be seen that the mechanisms of NK-Exos killing tumor cells and affecting their killing ability need to be explored (Table 3, Figure 4).
![]() |
Table 3 Role of NK Cells and DC Cells Derived Exosomes in Tumor Microenvironment |
![]() |
Figure 4 Effect of NK cell derived exosomes on tumor cells. |
DC Cells
DC cells are the most powerful specialized antigen presenting cells (APCs) in the body, which can efficiently absorb, process and present antigens. Immature DC have strong migration ability, mature DC can activate naive T cells effectively, which is the central link of initiating, regulating and maintaining immune response.103,104
The Effect of Exosomes Derived from DC Cells on T Cells
In addition to the activation of T cells by binding of MHC molecules and TCR on the surface of T cells, exosomes secreted by DC cells (DC-Exos) also play an important role in the activation of T cells. First, it was found that DC-Exos can promote the survival of naive CD4+ T cells in a manner that induces NF-κB activation through MHC-II binding to the TCR.105 Meanwhile, Elodie Segura et al showed that mature DC cell-derived exosomes are rich in MHC II, B7.2 and intercellular adhesion molecule-1 (ICAM-1), exosomes require MHC II and ICAM-1 to initiate naive CD4+ T cells, whereas B7.2 is not required.106 In turn, activated T cells recruit DC-Exos via leukocyte function-associated antigen 1(LFA-1).107 In addition, Xiang et al demonstrated that OVA protein-pulsed DC-Exos can be targeted for uptake by non-specific CD4+ T cells through ICAM-1/LFA −1 interactions; CD4+ T cells at this time are able to express an acquired exosome MHC I/OVA I peptide (pMHC I) complex and costimulatory CD40 and CD80 molecules, and can act as APC cells to stimulate specific CD8+ T cell proliferation, cytotoxic effects and anti-tumor immunity.108 Poly (I:C), as a ligand of TLR-3, plays an anti-cancer role by activating related signal pathways. Interestingly, it can also promote DC-Exos to induce CD8+T cell proliferation, cytotoxicity and strong tumor immune response to cervical cancer, indicating the potential of poly (I:C) in DC-Exos based vaccines to improve cervical cancer.109 In the process of DC-Exos promoting the function of T cells, B cells play an important role. Firstly, Susanne Gabrielsson et al found that DC-Exos can provide antigens for B cell activation, and in B cell signal deficient mice, exosomes cannot effectively induce B cell and T cell responses, proving that B cells play an important role in the process of exosomes inducing naive T cells to differentiate into Th1. At the same time, the research team also proved that B cells and CD4+T cells are crucial to the CD8+T cell response induced by DC-Exos110,111 (Table 3, Figure 5).
![]() |
Figure 5 Promoting effect of DC cell-derived exosomes on other immune cells. |
The Effect of DC Cell Derived Exosomes on NK Cells
Venkateswara Rao Simhadri et al found that DC cells release HLA-B-related transcription-3 positive exosomes, which can act as receptors to bind to ligands on NK cells to enhance natural killer function.112 Similarly, DC-Exos also overexpress IL-15Rα and NKG2D, which are the key to promote the proliferation and activation of NK cells, and can promote the secretion of IFN-γ by NK cells, enhance the tumor killing effect of NK cells.113 In addition, similar to DC, DC-Exos express TNF superfamily ligands (TNF, FasL and TRAIL) on their surfaces, which can trigger caspase activation in tumor cells to directly induce tumor cell apoptosis. TNF in exosomes can also interact with TNF receptor of NK cells to activate NK cells to secrete IFN-γ further kill tumor cells114 (Table 3, Figure 5).
Communication Between DC Cells
DC-Exos can induce bystander DC cell maturation by binding to bystander DC cells and cross-presenting TLR-L, increase the expression of tmTNF, and enhance the secretion of major pro-inflammatory and immunomodulatory cytokines to effectively stimulate the intense innate immune pro-inflammatory response and Th1 polarization.115 In addition to exosomes, the communication between DC cells can also take the form of synapses. The researchers first used ZsGreen fluorescent staining to trace the melanoma antigen protein of mice in vivo.116 It was found that the antigen in the tumor microenvironment was ingested by migratory dendritic cells (mDCs), packaged in the form of “vesicles” and entered the draining lymph nodes (TDLNs) with mDCs. Before being presented to T cells, the antigen would first be transmitted in DC of different subtypes.116 They found that mDCs entering tdLNs were closely connected with resident dendritic cells (rDCs) in tdLNs, and formed synapses. Vesicle transfer occurred in the absence of free exosomes, enabling antigen transmission between different DC cells. After this internal transmission is completed, different DCs will find corresponding T cells to complete antigen presentation. Further studies showed that this delivery mode also enhanced the downstream tumor antigen presentation cascade116 (Table 3, Figure 5).
MDSC Cells
MDSC is a group of immature bone marrow-derived cells that are activated and mobilized under tumor and other pathological conditions and have T cell inhibitory function.117 In terms of cell morphology, MDSC can be divided into two subgroups: monocyte like MDSC (M-MDSC) and granulocyte like MDSC (G-MDSC).118 Based on mass spectrometry proteomics and next-generation sequencing, the exosomes from MDSC (MDSC-Exo) were analyzed, and a large number of proteins and RNAs related to immunosuppressive function were identified, which provided different and complementary immunosuppressive functions compared with MDSC. This provides a basis for studying the tumor immunosuppressive effect of MDSC-Exos.119 Cancer stem cells are a kind of cancer cells with similar functions to stem cells. They have the ability of self-renewal and differentiation into cancer cells, and are considered to be closely related to the occurrence and development of tumors. In colorectal cancer, S100A9 is highly expressed in G-MDSC-Exos, which can not only promote the dryness and growth of colorectal cancer cells, but also promote the chemotaxis of G-MDSC to peripheral blood and colorectal tissues, thus weakening the anti-tumor immunity.120 Moreover, these effects are particularly significant in hypoxic environments. Therefore, researchers believe that respiratory hyperoxia may be an effective way to reduce the dryness of colorectal cancer cells, and the exosomal S100A9 from MDSCs may be an important marker to predict the occurrence and development of colorectal cancer.120 Similarly, in lung cancer, miR-143-3p increased in G-MDSC-Exos and down regulated membrane protein 2B (ITM2B) to activate PI3K/Akt signaling pathway to promote tumor proliferation.121 MDSC-Exos can also target immune cells, which can increase the level of reactive oxygen species and stimulate Fas/FasL pathway in CD8+T cells to promote CD8+T cell apoptosis, thus exerting immunosuppressive effects.122 In conclusion, MDSC-Exos play a significant role in promoting tumor cell proliferation, angiogenesis, and immunosuppression. Perhaps targeting their generation can become one of the adjuvant tumor therapies (Table 4).
![]() |
Table 4 The Role of Exosomes from MDSC, B Cells, Mast Cells and Neutrophils in the Tumor Microenvironment |
B Cells
In addition to producing antibodies, exosomes secreted by B cells (B-Exos) also play an important role in tumor immunity. And some studies have shown that CD40 and IL-4 receptors can stimulate B cells to secrete more exosomes.123,124 At the same time, as APC, the pMHC II complex carried on the surface of B cells can also induce the secretion of exosomes of B cells to increase after binding with TCR on the surface of CD4+T cells.123,124 These studies have laid the foundation for B-Exos to play a role in the tumor microenvironment. Next, we will explore how it plays a role and what its final effect is. B cell derived lymphoid cell line (LCL) can secrete MHCII+ FasL+ exosomes and induce CD4+T cell apoptosis.125 Similarly, B cells can produce CD19+ exosomes, which can highly express CD39 and CD73. CD39 (exonucleoside triphosphate diphosphate hydrolase) can hydrolyze ATP and ADP produced by tumor cells to AMP. CD73 can hydrolyze amp to adenosine, which can inhibit the proliferation and killing ability of CD8+T cells. They also found that hypoxia increased the expression of Rab27a, a key protein for exosome production in B cells, which in turn increased exosome production.126 Ovarian cancer, mainly high-grade serous ovarian cancer (HGSC) can be divided into four subtypes: immune response subtype, differentiation subtype, proliferation subtype and mesenchymal subtype, the prognosis of mesenchymal subtypes is relatively poor.127 The study found that the abundance of B cell subsets correlated closely with the number of mesenchymal subtypes of ovarian cancer cells, and plasma cells can increase the expression of junctional adhesion molecule B on non-mesenchymal subtype ovarian cancer cells by secreting exosomes encasing miR-330-3p, thereby promoting their transformation into mesenchymal128 (Table 4).
Mast Cells
Mast cells are one of the main immune cells in human body, the most important structural feature of mast cells is that there are a lot of basophilic particles in the cells. Among them, histamine has an important effect on the proliferation, migration and invasion of tumor cells, while VEGF, FGF, MMP, trypsin and chymotrypsin may play an important role in different stages of tumor angiogenesis.129 Early experiments showed that mast cell-derived exosomes (mast-Exos) contained immune-related molecules, such as MHC II, CD86, LFA-1 and ICAM-1, which induced B and T lymphocyte proliferation and cytokine production.130 In addition, they also found that the mast-Exos contain exogenous Ags and endogenous heat shock proteins, which can induce specific IgG1 and IgG2a antibody responses in mice, and these exosomes can effectively promote the maturation of DC cells, play the antigen presentation function, present specific antigen to T cells, and activate T cells.131 OX40L expressed on the surface of mast-Exos can combine with OX40 on the surface of CD4+T cells to promote the proliferation of CD4+T cells and differentiation into Th cells.132 These studies have proved that mast-Exos play an important role in tumor immunity as a tool of intercellular communication. Mast-Exos can also transfer KIT protein to lung adenocarcinoma cells and activate PI3K/AKT signaling pathway to promote tumor cell proliferation133 (Table 4).
Neutrophils
Neutrophils participate in different stages of tumorigenesis and development, including tumorigenesis, proliferation and metastasis. The neutrophil population in the tumor microenvironment shows heterogeneous phenotype and functional diversity, and plays a dual role of tumor promotion or tumor inhibition in the tumor microenvironment.134,135 However, there are few studies on neutrophil-derived exosomes. Recent study has shown that neutrophils transformed to N2 in a STAT3-dependent manner are recruited in the brain metastasis pre niche under the action of nicotine. The high expression of miR-4466 in the secreted exosomes can promote the stem and metabolic transformation of tumor cells through the SKI/SOX2/CPT1A axis in brain tumor cells, thus providing the possibility for metastasis.136 Neutrophils are the most abundant immune cells in the circulation, and their secreted exosomes may play more roles in the tumor microenvironment, which may be a hot cell in future research and drug development (Table 4).
The Potential Clinical Application of Exosomes Derived from Immune Cells
The Role of Engineered DC-Exos in Anti-Tumor
In recent years, the role of DC tumor vaccine in tumor therapy has gradually become a research hotspot. DC tumor vaccines are made by sensitizing DC cells to tumor cell DNA, RNA, tumor Lysis, tumor antigen protein polypeptides. The powerful presentation function of DCs is utilized to activate T cell immune responses in patients, thus play a role in the treatment of cancer.137,138 In 1998, Amigorena et al validated the surface expression of MHC I and MHC II, as well as T cell co-stimulatory molecules, on DC-Exos after tumor peptide pulses in animal experiments. It can induce specific cytotoxic T cell responses in vivo to exert anti-tumor effects.139 This opens the way for DC-Exos to be used as tumor vaccines. In hepatocellular carcinoma, researchers extracted DC-Exos expressing AFP (DEXAFP) and demonstrated that it can induce potent anti-tumor immune responses in ectopic or orthotopic HCC mice, the immunosuppressive microenvironment of the tumor was improved, including increased infiltration of CD8+CTL, high levels of IFN-γ and IL-2, decreased levels of the immunosuppressive cytokines IL-10, TGF-β and Treg cells. This study provides evidence that DC-Exos can be used as a cell-free vaccine for HCC immunotherapy and is effective in controlling HCC progression in patients.140 Microwave Ablation (MWA), a form of thermal ablation, is widely used in the treatment of HCC, where the combination of MWA and DC-Exos significantly inhibited the growth of tumor and effectively improved the tumor immune microenvironment.141 In a mouse model of melanoma, the researchers used the TLR-3 ligand poly (I: C), TLR-4 ligand LPS, or TLR-9 ligand CpG-B to stimul DC cells when preparing DC-Exos. When Poly (I: C) was mixed with ovalbumin (OVA) for the preparation of exosomes, the resulting DC-Exos vaccine was able to strongly stimulate the proliferation of specific CD8+ T and CD4+ T cells and promote their effector function.142 In NSCLC, a Phase I study recruited pre-treated stage IIIB NSCLC patients with MAGE expression and generated DC-Exos loaded with MAGE antigens demonstrating its safety and efficacy in NSCLC patients.143 A Phase II trial tested the clinical efficacy of IFN-γ-DC-Exos loaded with MHC I and II as post-chemotherapy immunotherapy in patients with inoperable NSCLC, and found that IFN-γ-DC-Exos enhanced NKp30-dependent NK cell function, but had no effect on specific T-cell responses.144
ICB has recently played an important role in the immunotherapy of tumors, but its efficacy in most tumors is limited, which requires a combination of multiple therapeutic approaches. Li et al engineered DC-Exos with anti-CD3 and anti-EGFR to prepare DC-Exos as a “Bridge” between tumor cells and activated T cells. DC-Exos can not only promote T cell proliferation and differentiation through MHC-antigenic peptide complex and CD86 costimulatory molecules, but also mediate the binding of activated T cells to cancer cells.145 In addition, DC-Exos showed excellent anti-tumor activity in mouse tumor model, and effectively inhibited tumor recurrence and metastasis. In particular, the researchers found that DC-Exos enhanced the anti-tumor immune response and promoted the expression of PD-L1, an immune checkpoint in tumor tissue. Therefore, combined anti-PD-L1 ICB therapy effectively reduced the immune escape of tumor cells and further enhanced the therapeutic effect of DC-Exos on solid tumors.145 In addition, Jong Oh Kim et al also prepared a CTLA-4 antibody-modified DC-Exos, which is able to target T cells, effectively induce T cell activation, and increase the ratio of effector T cells/Tregs in tumor tissue, induced a robust tumor-specific T cell killing response and improved tumor homing of effector T cells, ultimately significantly suppressing tumor growth.146 These results suggest that the combination of DC-Exos and immune checkpoint therapy may play a better role in cancer therapy.
The Role of Engineered Macrophage-Derived Exosomes in Anti-Tumor
M1 macrophages play a pro-inflammatory role in the tumor microenvironment, and based on their anti-tumor properties, most exosomes of engineered macrophages are derived from M1-type. After the M1-Exos were extracted, the researchers found, by tracing techniques, that these exosomes were taken up by macrophages and DC cells in mice and promoted the release of pro-inflammatory factors. At the same time, these exosomes enhanced the activity of the lipid-calcium phosphate nanoparticle-encapsulated Trp2 vaccine and induced a stronger antigen-specific cytotoxic T-cell response.147 Chen et al co-cultured M1-Exos with M0 macrophages and found that exosomes could activate the NF-κB pathway of M0 macrophages and promote the polarization of M0 toward M1, in addition, a lot of inflammatory cytokines (IL-6, IL-12 and TNF-α) were released to establish the local inflammatory microenvironment.148 On this basis, they used acoustic technology to make exosomes loaded with paclitaxel, and in vitro cytotoxicity experiments found that the same dose of paclitaxel after exosome-loaded tumor cells increased inhibition. The in vivo 4T1 tumor-bearing mice experiment further proved that the therapeutic effect of paclitaxel was more significant after the exosome was loaded with paclitaxel.148 Similarly, Wang et al favored electroporation of Cisplatin into M1-Exos, which significantly enhanced the proliferation inhibition and apoptosis rate of lung cancer cells.149 In addition to simply encapsulating chemotherapeutic drugs, Wei et al constructed M1-derived extracellular vesicles (CCA-M1EV) that were treated with two hydrophobic agents (chemical excitation source CPPO and photosensitizer CE6) to label the membrane of EVs, AQ4N drug precursors were loaded into the vesicles. After intravenous injection, the inherent physicochemical properties of CCA-M1EV allow it to cross the blood-brain barrier and modulate the immunosuppressive tumor microenvironment by promoting M2 to M1 polarization, thereby increasing hydrogen peroxide levels.150 In addition, hydrogen peroxide reacts with CPPO to produce chemical energy that can be used for the activation of Ce6, and this process also generates large amounts of reactive oxygen species to achieve chemo-stimulated photodynamic therapy (CDT). As this reaction consumes oxygen, it also causes the non-toxic AQ4N to turn into toxic AQ4 for chemotherapy. Therefore, CCA-M1EV achieves the synergistic effect of immunoregulation, CDT and hypoxia-activated chemotherapy, and plays a powerful therapeutic role.150
M2 macrophages play an important role in promoting tumor growth in the tumor microenvironment, and M1-Exos also play an important role in promoting repolarization of M2 macrophages. Lee et al constructed IL4R-Exo(si/mi) and transfected M1-Exos with NF-B p50 siRNA and miR-511-3p to enhance M1 polarization, they were surface modified with IL4R binding peptide IL4RPep-1 to target the IL4 receptor of TAMs. In M2 macrophages, IL4R-EXO (si/mi) was taken up by M2 macrophages. The systemic administration of IL4R-Exo(si/mi) could effectively inhibit tumor growth, down-regulate target genes, decrease the levels of M2 cytokines and immunosuppressive cells, and increase the levels of M1 cytokines and immunostimulatory cells.151 On this basis, the researchers also explored whether repolarization of macrophages by exosomes could enhance the anti-cancer efficacy of aPD-L1. Exosome treatment induced M2 macrophages to polarize into M1 macrophages in vitro and in vivo. Exosomes were injected intravenously into tumor-bearing mice to inhibit tumor growth. Importantly, the combination of simultaneous injection of M1NV and aPD-L1 further reduced tumor size compared with M1NV or aPD-L1 alone.152 Interestingly, the researchers introduced nuclei isolated from tumor cells into activated M1 macrophages and then produced biologically reprogrammed macrophage-tumor chimeric exosomes (aMT-exos). This exosome can play a dual role in both increasing T cell production and regulating the immunosuppressive tumor microenvironment.153
The Role of Engineered NK-Exos in Anti-Tumor
The anti-apoptotic protein BCL-2 is highly expressed in multiple tumors and is associated with poor prognosis, and Melanie M ärklin et al used lentiviral transfection of the NK cell line NK92MI to express BCL-2 siRNA (siBCL-2) and load it into exosomes, targeting BCL-2 via siBCL-2 NK-Exos resulted in enhanced apoptosis of breast cancer cells without affecting other cells.154 Similarly, NK-Exos coated with paclitaxel (PTX-NK-Exos) were successfully prepared by electroporation, it can be taken up by human breast cancer cells to increase the apoptosis rate of MCF-7 cells by inducing Bax and caspase-3 in the tumor cell apoptosis signaling pathway.155 The researchers also used NK-Exos and their biomimetic core-shell structure nanoparticles (NNs) to target tumors. NNs self-assemble with dendritic polymer cores loaded with therapeutic miRNA and hydrophilic NK-Exos shells, and NK-Exos directs NNs to tumors and interacts with the plasma membrane of target cells via endocytosis/fusion or FasL/Fas. Finally, therapeutic miRNA are released and regulate the transcriptome batch or gene of the cell, exerting a combined antitumor effect with NK-Exos.156
The Role of Exosomes Derived from Other Engineered Immune Cells in Anti-Tumor
In Zhang et al’s study, they first demonstrated that neutrophil exosomes can induce tumor cell apoptosis by delivering cytotoxic proteins and activating caspase signaling pathways. Furthermore, neutrophil exosomes modified superparamagnetic iron oxide nanoparticles (SPIONs) were also prepared to achieve more efficient tumor targeted therapy. Studies have shown that superparamagnetic neutrophil exosomes loaded with chemotherapy drug doxorubicin (DOX) can be highly targeted and enriched at tumor sites under the action of external magnetic field, playing a powerful role in inhibiting tumor proliferation.157 Interestingly, researchers have also developed a CD8+ T-cell-derived nanovesicles (TCNVs), which are nanoscale vesicles generated by the continuous extrusion of cells, which are more productive than naturally secreted exosomes and contain more RNA and protein. Cytotoxic T cell membrane proteins (such as PD-1 and TGF-β receptors: TGF-βR) and intracellular granzyme B were preserved in TCNVs. PD-1 and TGF-βR can interact with PD-L1 and TGF-β in the TME on cancer cells, respectively, preventing PD-L1 or TGF-β from leading to depletion of CD8+ T cells. In addition, TCNVs can directly induce cancer cell apoptosis by delivering granzyme B to cancer cells.158
In the future, there will be many exosomes derived from immune cells, which will be gradually perfected by engineering to play a role in tumor therapy, in addition, we need to conduct a large number of cells, animal and clinical trials to verify their efficacy.
Conclusion and Outlook
The role of tumor-derived exosomes in the microenvironment, as important signal and material transport vehicles, has become a research hotspot in recent years, and drug delivery systems that target its biocompatibility have also played a role in cancer treatment. Cell-to-cell communication is never one-way, and in addition to tumor cells, other stromal cell-derived exosomes in the microenvironment, especially immune cells, cannot be ignored. The double-edged sword effect and mechanism of exosomes derived from different immune cells in tumor microenvironment were discussed in this paper, and the roles of these exosomes in tumor therapy were summarized. However, the role of these exosomes as drug delivery tools for cancer therapy remains to be explored:
- The exosomes derived from different immune cells in different populations are heterogeneous. How to overcome the heterogeneity and seek the exosomes that can play the most important role.
- Tumor heterogeneity is also enormous, for different tumors to select specific to play the most effective exosomes.
- It is important to ensure that engineered exosomes deliver targeted tumor-killing effects without affecting other cells.
- Tumor immune microenvironment is complex, whether these exosomes can overcome the role of immunosuppressive factors and immunosuppressive cells in the microenvironment, so that immune killer cells play a greater role.
With the development of immunotherapy, drug delivery tools based on exosomes derived from immune cells may become another important breakthrough in immunotherapy.
Abbreviations
APCs, Antigen presenting cells; aTreg, Adaptive regulatory T cells; CAR-T, Chimeric antigen receptor T-cell immunotherapy; CAFs, Cancer-related fibroblasts; CDKN1B, Cyclin-dependent kinase inhibitor 1B; CDT, Chemo-stimulated photodynamic therapy; DCs, Dendritic cells; DR5, Death receptor 5; GM-CSF, Granulocyte-macrophage colony stimulating factor; G-MDSC, Granulocyte like MDSC; HBsAb, Hepatitis B surface antibody; HBsAg, Hepatitis B surface antigen; HGSC, High-grade serous ovarian cancer; ICB, Immune checkpoint blocking; ICAM-1, Intercellular adhesion molecule-1; ILVs, Intraluminal vesicles; LCL, Lymphoid cell line; LFA-1, Leukocyte function-associated antigen 1; LPS, Lipopolysaccharide; mDCs, Migratory dendritic cells; M-MDSC, Monocyte like MDSC; MVBs, Multivesicular bodies; MSCs, Mesenchymal stem cells; nTreg, Natural regulatory T cells; NKT, Natural killer T cells; NPC, Nasopharyngeal carcinoma; OSCC, Oral squamous-cell carcinoma cells; PD-1, Programmed Cell Death 1; PGE2, Prostaglandin E2; rDCs, Resident dendritic cells; STK16, Serine/threonine kinase 16; Treg, Regulatory T cells; TGF-β, Transforming growth factor-β; UCEC, Uterine corpus endometrial cancer; ZC3H12B, Zinc-finger-type-containing 12B.
Ethics Approval
The authors declare that their participation in writing this review as well as its publication is voluntary.
Author Contributions
All authors made a significant contribution to the work reported, whether that is in the conception, study design, execution, acquisition of data, analysis and interpretation, or in all these areas; took part in drafting, revising or critically reviewing the article; gave final approval of the version to be published; have agreed on the journal to which the article has been submitted; and agree to be accountable for all aspects of the work. Shiyang Wang: Conceptualization, Writing – Original Draft, Writing – Review & Editing. Yue Shi: Writing – Review & Editing, Supervision.
Funding
There is no funding to report.
Disclosure
No conflict of interest can be disclosed. The authors declare that they have no competing interests.
References
1. Kalluri R, LeBleu VS. The biology, function, and biomedical applications of exosomes. Science. 2020;367(6478). doi:10.1126/science.aau6977
2. Liu J, Peng X, Yang S, et al. Extracellular vesicle PD-L1 in reshaping tumor immune microenvironment: biological function and potential therapy strategies. CCS. 2022;20(1):14. doi:10.1186/s12964-021-00816-w
3. Olejarz W, Kubiak-Tomaszewska G, Chrzanowska A, Lorenc T. Exosomes in angiogenesis and anti-angiogenic therapy in cancers. Int J Mol Sci. 2020;21(16). doi:10.3390/ijms21165840
4. van Niel G, Carter DRF, Clayton A, Lambert DW, Raposo G, Vader P. Challenges and directions in studying cell-cell communication by extracellular vesicles. Nat Rev Mol Cell Biol. 2022;23(5):369–382. doi:10.1038/s41580-022-00460-3
5. Cheng L, Hill AF. Therapeutically harnessing extracellular vesicles. Nat Rev Drug Discov. 2022;21(5):379–399. doi:10.1038/s41573-022-00410-w
6. Huang R, Li X, He Y, et al. Recent advances in CAR-T cell engineering. J Hematol Oncol. 2020;13(1):86. doi:10.1186/s13045-020-00910-5
7. Peng M, Mo Y, Wang Y, et al. Neoantigen vaccine: an emerging tumor immunotherapy. Molecular Cancer. 2019;18(1):128. doi:10.1186/s12943-019-1055-6
8. Morad G, Helmink BA, Sharma P, Wargo JA. Hallmarks of response, resistance, and toxicity to immune checkpoint blockade. Cell. 2021;184(21):5309–5337. doi:10.1016/j.cell.2021.09.020
9. Cocozza F, Grisard E, Martin-Jaular L, Mathieu M, Thery C. SnapShot: extracellular vesicles. Cell. 2020;182(1):262–262e1. doi:10.1016/j.cell.2020.04.054
10. Hessvik NP, Llorente A. Current knowledge on exosome biogenesis and release. Cell Mol Life Sci. 2018;75(2):193–208. doi:10.1007/s00018-017-2595-9
11. Stuffers S, Sem Wegner C, Stenmark H, Brech A. Multivesicular endosome biogenesis in the absence of ESCRTs. Traffic. 2009;10(7):925–937. doi:10.1111/j.1600-0854.2009.00920.x
12. Pegtel DM, Gould SJ. Exosomes. Annu Rev Biochem. 2019;88:487–514. doi:10.1146/annurev-biochem-013118-111902
13. Wei D, Zhan W, Gao Y, et al. RAB31 marks and controls an ESCRT-independent exosome pathway. Cell Research. 2021;31(2):157–177. doi:10.1038/s41422-020-00409-1
14. Kalluri R. The biology and function of exosomes in cancer. J Clin Invest. 2016;126(4):1208–1215. doi:10.1172/JCI81135
15. Keerthikumar S, Chisanga D, Ariyaratne D, et al. ExoCarta: a web-based compendium of exosomal cargo. Journal of Molecular Biology. 2016;428(4):688–692. doi:10.1016/j.jmb.2015.09.019
16. Wang B, Mao JH, Wang BY, et al. Exosomal miR-1910-3p promotes proliferation, metastasis, and autophagy of breast cancer cells by targeting MTMR3 and activating the NF-κB signaling pathway. Cancer Letters. 2020;489:87–99. doi:10.1016/j.canlet.2020.05.038
17. Zeng Z, Li Y, Pan Y, et al. Cancer-derived exosomal miR-25-3p promotes pre-metastatic niche formation by inducing vascular permeability and angiogenesis. Nat Commun. 2018;9(1):5395. doi:10.1038/s41467-018-07810-w
18. Yang C, Wu S, Mou Z, et al. Exosome-derived circTRPS1 promotes malignant phenotype and CD8+ T cell exhaustion in bladder cancer microenvironments. Molecul Ther. 2022;30(3):1054–1070. doi:10.1016/j.ymthe.2022.01.022
19. Chen G, Huang AC, Zhang W, et al. Exosomal PD-L1 contributes to immunosuppression and is associated with anti-PD-1 response. Nature. 2018;560(7718):382–386. doi:10.1038/s41586-018-0392-8
20. Bae YH, Park K. Advanced drug delivery 2020 and beyond: perspectives on the future. Adv Drug Deliv Rev. 2020;158:4–16. doi:10.1016/j.addr.2020.06.018
21. Mi P, Cabral H, Kataoka K. Ligand-installed nanocarriers toward precision therapy. Adv Mater. 2020;32(13):e1902604. doi:10.1002/adma.201902604
22. Elsharkasy OM, Nordin JZ, Hagey DW, et al. Extracellular vesicles as drug delivery systems: why and how? Adv Drug Deliv Rev. 2020;159:332–343. doi:10.1016/j.addr.2020.04.004
23. Liang Y, Duan L, Lu J, Xia J. Engineering exosomes for targeted drug delivery. Theranostics. 2021;11(7):3183–3195. doi:10.7150/thno.52570
24. Thakur A, Ke X, Chen YW, et al. The mini player with diverse functions: extracellular vesicles in cell biology, disease, and therapeutics. Protein Cell. 2022;13(9):631–654. doi:10.1007/s13238-021-00863-6
25. Thakur A, Parra DC, Motallebnejad P, Brocchi M, Chen HJ. Exosomes: small vesicles with big roles in cancer, vaccine development, and therapeutics. Bioact Mater. 2022;10:281–294. doi:10.1016/j.bioactmat.2021.08.029
26. Zhou W, Zhou Y, Chen X, et al. Pancreatic cancer-targeting exosomes for enhancing immunotherapy and reprogramming tumor microenvironment. Biomaterials. 2021;268:120546. doi:10.1016/j.biomaterials.2020.120546
27. Huang L, Rong Y, Tang X, et al. Engineered exosomes as an in situ DC-primed vaccine to boost antitumor immunity in breast cancer. Molecular Cancer. 2022;21(1):45. doi:10.1186/s12943-022-01515-x
28. Kim MS, Haney MJ, Zhao Y, et al. Development of exosome-encapsulated paclitaxel to overcome MDR in cancer cells. Nanomedicine. 2016;12(3):655–664. doi:10.1016/j.nano.2015.10.012
29. Saari H, Lazaro-Ibanez E, Viitala T, Vuorimaa-Laukkanen E, Siljander P, Yliperttula M. Microvesicle- and exosome-mediated drug delivery enhances the cytotoxicity of Paclitaxel in autologous prostate cancer cells. J Control Release. 2015;220(Pt B):727–737. doi:10.1016/j.jconrel.2015.09.031
30. Wei H, Chen J, Wang S, et al. A nanodrug consisting of doxorubicin and exosome derived from mesenchymal stem cells for osteosarcoma treatment in vitro. Int J Nanomedicine. 2019;14:8603–8610. doi:10.2147/IJN.S218988
31. Thakur A, Sidu RK, Zou H, Alam MK, Yang M, Lee Y. Inhibition of glioma cells’ proliferation by doxorubicin-loaded exosomes via microfluidics. International Journal of Nanomedicine. 2020;15:8331–8343. doi:10.2147/ijn.S263956
32. Thakur A, Johnson A, Jacobs E, et al. Energy sources for exosome communication in a cancer microenvironment. Cancers. 2022;14(7). doi:10.3390/cancers14071698
33. Gong N, Sheppard NC, Billingsley MM, June CH, Mitchell MJ. Nanomaterials for T-cell cancer immunotherapy. Nat Nanotechnol. 2021;16(1):25–36. doi:10.1038/s41565-020-00822-y
34. Speiser DE, Ho PC, Verdeil G. Regulatory circuits of T cell function in cancer. Nat Rev Immunol. 2016;16(10):599–611. doi:10.1038/nri.2016.80
35. van der Leun AM, Thommen DS, Schumacher TN. CD8(+) T cell states in human cancer: insights from single-cell analysis. Nat Rev Cancer. 2020;20(4):218–232. doi:10.1038/s41568-019-0235-4
36. Zhang N, Bevan MJ. CD8(+) T cells: foot soldiers of the immune system. Immunity. 2011;35(2):161–168. doi:10.1016/j.immuni.2011.07.010
37. Reina-Campos M, Scharping NE, Goldrath AW. CD8(+) T cell metabolism in infection and cancer. Nat Rev Immunol. 2021;21(11):718–738. doi:10.1038/s41577-021-00537-8
38. Timaner M, Tsai KK, Shaked Y. The multifaceted role of mesenchymal stem cells in cancer. Semin Cancer Biol. 2020;60:225–237. doi:10.1016/j.semcancer.2019.06.003
39. De Wever O, Van Bockstal M, Mareel M, Hendrix A, Bracke M. Carcinoma-associated fibroblasts provide operational flexibility in metastasis. Semin Cancer Biol. 2014;25:33–46. doi:10.1016/j.semcancer.2013.12.009
40. Seo N, Shirakura Y, Tahara Y, et al. Activated CD8(+) T cell extracellular vesicles prevent tumour progression by targeting of lesional mesenchymal cells. Nat Commun. 2018;9(1):435. doi:10.1038/s41467-018-02865-1
41. Zhou WJ, Zhang J, Xie F, et al. CD45RO(-)CD8(+) T cell-derived exosomes restrict estrogen-driven endometrial cancer development via the ERβ/miR-765/PLP2/Notch axis. Theranostics. 2021;11(11):5330–5345. doi:10.7150/thno.58337
42. Cai Z, Yang F, Yu L, et al. Activated T cell exosomes promote tumor invasion via Fas signaling pathway. J Immunol. 2012;188(12):5954–5961. doi:10.4049/jimmunol.1103466
43. Wang X, Shen H, He Q, Tian W, Xia A, Lu XJ. Exosomes derived from exhausted CD8+ T cells impaired the anticancer function of normal CD8+ T cells. Journal of Medical Genetics. 2019;56(1):29–31. doi:10.1136/jmedgenet-2018-105439
44. Silva-Santos B, Serre K, Norell H. γδ T cells in cancer. Nat Rev Immunol. 2015;15(11):683–691. doi:10.1038/nri3904
45. Kabelitz D, Serrano R, Kouakanou L, Peters C, Kalyan S. Cancer immunotherapy with γδ T cells: many paths ahead of us. Cell Mol Immunol. 2020;17(9):925–939. doi:10.1038/s41423-020-0504-x
46. Wang X, Xiang Z, Liu Y, et al. Exosomes derived from Vδ2-T cells control Epstein-Barr virus-associated tumors and induce T cell antitumor immunity. Science Translational Medicine. 2020;12(563). doi:10.1126/scitranslmed.aaz3426
47. Wang X, Zhang Y, Mu X, et al. Exosomes derived from γδ-T cells synergize with radiotherapy and preserve antitumor activities against nasopharyngeal carcinoma in immunosuppressive microenvironment. J Immunother Cancer. 2022;10(2). doi:10.1136/jitc-2021-003832
48. Li L, Lu S, Liang X, et al. γδTDEs: an efficient delivery system for miR-138 with anti-tumoral and immunostimulatory roles on oral squamous cell carcinoma. Mol Ther Nucleic Acids. 2019;14:101–113. doi:10.1016/j.omtn.2018.11.009
49. Oh DY, Fong L. Cytotoxic CD4(+) T cells in cancer: expanding the immune effector toolbox. Immunity. 2021;54(12):2701–2711. doi:10.1016/j.immuni.2021.11.015
50. Borst J, Ahrends T, Bąbała N, Melief CJM, Kastenmüller W. CD4(+) T cell help in cancer immunology and immunotherapy. Nat Rev Immunol. 2018;18(10):635–647. doi:10.1038/s41577-018-0044-0
51. Zhang H, Xie Y, Li W, Chibbar R, Xiong S, Xiang J. CD4(+) T cell-released exosomes inhibit CD8(+) cytotoxic T-lymphocyte responses and antitumor immunity. Cell Mol Immunol. 2011;8(1):23–30. doi:10.1038/cmi.2010.59
52. Lu J, Wu J, Xie F, et al. CD4(+) T Cell-released extracellular vesicles potentiate the efficacy of the HBsAg vaccine by enhancing B cell responses. Adv Sci. 2019;6(23):1802219. doi:10.1002/advs.201802219
53. Wing JB, Tanaka A, Sakaguchi S. Human FOXP3(+) regulatory T cell heterogeneity and function in autoimmunity and cancer. Immunity. 2019;50(2):302–316. doi:10.1016/j.immuni.2019.01.020
54. Sakaguchi S, Mikami N, Wing JB, Tanaka A, Ichiyama K, Ohkura N. Regulatory T cells and human disease. Annu Rev Immunol. 2020;38:541–566. doi:10.1146/annurev-immunol-042718-041717
55. Xie Y, Zhang X, Zhao T, Li W, Xiang J. Natural CD8+25+ regulatory T cell-secreted exosomes capable of suppressing cytotoxic T lymphocyte-mediated immunity against B16 melanoma. Biochemical and Biophysical Research Communications. 2013;438(1):152–155. doi:10.1016/j.bbrc.2013.07.044
56. Aiello S, Rocchetta F, Longaretti L, et al. Extracellular vesicles derived from T regulatory cells suppress T cell proliferation and prolong allograft survival. Sci Rep. 2017;7(1):11518. doi:10.1038/s41598-017-08617-3
57. Smyth LA, Ratnasothy K, Tsang JY, et al. CD73 expression on extracellular vesicles derived from CD4+ CD25+ Foxp3+ T cells contributes to their regulatory function. European Journal of Immunology. 2013;43(9):2430–2440. doi:10.1002/eji.201242909
58. Tung SL, Boardman DA, Sen M, et al. Regulatory T cell-derived extracellular vesicles modify dendritic cell function. Sci Rep. 2018;8(1):6065. doi:10.1038/s41598-018-24531-8
59. Rosenberg SA, Restifo NP. Adoptive cell transfer as personalized immunotherapy for human cancer. Science. 2015;348(6230):62–68. doi:10.1126/science.aaa4967
60. Porter DL, Levine BL, Kalos M, Bagg A, June CH. Chimeric antigen receptor-modified T cells in chronic lymphoid leukemia. N Engl J Med. 2011;365(8):725–733. doi:10.1056/NEJMoa1103849
61. Fu W, Lei C, Liu S, et al. CAR exosomes derived from effector CAR-T cells have potent antitumour effects and low toxicity. Nat Commun. 2019;10(1):4355. doi:10.1038/s41467-019-12321-3
62. Morello A, Sadelain M, Adusumilli PS. Mesothelin-targeted CARs: driving T cells to solid tumors. Cancer Discov. 2016;6(2):133–146. doi:10.1158/2159-8290.Cd-15-0583
63. Yang P, Cao X, Cai H, et al. The exosomes derived from CAR-T cell efficiently target mesothelin and reduce triple-negative breast cancer growth. Cell Immunol. 2021;360:104262. doi:10.1016/j.cellimm.2020.104262
64. Sun C, Mezzadra R, Schumacher TN. Regulation and Function of the PD-L1 Checkpoint. Immunity. 2018;48(3):434–452. doi:10.1016/j.immuni.2018.03.014
65. Qiu Y, Yang Y, Yang R, et al. Activated T cell-derived exosomal PD-1 attenuates PD-L1-induced immune dysfunction in triple-negative breast cancer. Oncogene. 2021;40(31):4992–5001. doi:10.1038/s41388-021-01896-1
66. Bagchi S, Yuan R, Engleman EG. Immune checkpoint inhibitors for the treatment of cancer: clinical impact and mechanisms of response and resistance. Annual Review of Pathology. 2021;16:223–249. doi:10.1146/annurev-pathol-042020-042741
67. Guyon N, Garnier D, Briand J, et al. Anti-PD1 therapy induces lymphocyte-derived exosomal miRNA-4315 release inhibiting Bim-mediated apoptosis of tumor cells. Cell Death Dis. 2020;11(12):1048. doi:10.1038/s41419-020-03224-z
68. Wynn TA, Chawla A, Pollard JW. Macrophage biology in development, homeostasis and disease. Nature. 2013;496(7446):445–455. doi:10.1038/nature12034
69. Locati M, Curtale G, Mantovani A. Diversity, mechanisms, and significance of macrophage plasticity. Annual Review of Pathology. 2020;15:123–147. doi:10.1146/annurev-pathmechdis-012418-012718
70. Mehla K, Singh PK. Metabolic regulation of macrophage polarization in cancer. Trends Cancer. 2019;5(12):822–834. doi:10.1016/j.trecan.2019.10.007
71. Anderson NR, Minutolo NG, Gill S, Klichinsky M. Macrophage-based approaches for cancer immunotherapy. Cancer Res. 2021;81(5):1201–1208. doi:10.1158/0008-5472.Can-20-2990
72. Zhang Q, Fan Z, Zhang L, You Q, Wang L. Strategies for targeting serine/threonine protein phosphatases with small molecules in cancer. Journal of Medicinal Chemistry. 2021;64(13):8916–8938. doi:10.1021/acs.jmedchem.1c00631
73. Wang X, Huang R, Lu Z, Wang Z, Chen X, Huang D. Exosomes from M1-polarized macrophages promote apoptosis in lung adenocarcinoma via the miR-181a-5p/ETS1/STK16 axis. Cancer Science. 2022;113(3):986–1001. doi:10.1111/cas.15268
74. Li Z, Suo B, Long G, et al. Exosomal miRNA-16-5p derived from M1 macrophages enhances T cell-dependent immune response by regulating PD-L1 in gastric cancer. Front Cell Dev Biol. 2020;8:572689. doi:10.3389/fcell.2020.572689
75. Cianciaruso C, Beltraminelli T, Duval F, et al. Molecular profiling and functional analysis of macrophage-derived tumor extracellular vesicles. Cell Rep. 2019;27(10):3062–3080.e11. doi:10.1016/j.celrep.2019.05.008
76. Jiang H, Zhou L, Shen N, et al. M1 macrophage-derived exosomes and their key molecule lncRNA HOTTIP suppress head and neck squamous cell carcinoma progression by upregulating the TLR5/NF-κB pathway. Cell Death Dis. 2022;13(2):183. doi:10.1038/s41419-022-04640-z
77. Qian BZ, Pollard JW. Macrophage diversity enhances tumor progression and metastasis. Cell. 2010;141(1):39–51. doi:10.1016/j.cell.2010.03.014
78. De Palma M, Lewis CE. Macrophage regulation of tumor responses to anticancer therapies. Cancer Cell. 2013;23(3):277–286. doi:10.1016/j.ccr.2013.02.013
79. Chu IM, Hengst L, Slingerland JM. The Cdk inhibitor p27 in human cancer: prognostic potential and relevance to anticancer therapy. Nat Rev Cancer. 2008;8(4):253–267. doi:10.1038/nrc2347
80. Li X, Tang M. Exosomes released from M2 macrophages transfer miR-221-3p contributed to EOC progression through targeting CDKN1B. Cancer Med. 2020;9(16):5976–5988. doi:10.1002/cam4.3252
81. Yin Z, Ma T, Huang B, et al. Macrophage-derived exosomal microRNA-501-3p promotes progression of pancreatic ductal adenocarcinoma through the TGFBR3-mediated TGF-β signaling pathway. CR. 2019;38(1):310. doi:10.1186/s13046-019-1313-x
82. Mi X, Xu R, Hong S, Xu T, Zhang W, Liu M. M2 macrophage-derived exosomal lncRNA AFAP1-AS1 and MicroRNA-26a affect cell migration and metastasis in esophageal cancer. Mol Ther Nucleic Acids. 2020;22:779–790. doi:10.1016/j.omtn.2020.09.035
83. Song L, Luan B, Xu Q, Shi R, Wang X. microRNA-155-3p delivered by M2 macrophages-derived exosomes enhances the progression of medulloblastoma through regulation of WDR82. J Transl Med. 2022;20(1):13. doi:10.1186/s12967-021-03156-y
84. Wenes M, Shang M, Di Matteo M, et al. Macrophage Metabolism controls tumor blood vessel morphogenesis and metastasis. Cell Metabolism. 2016;24(5):701–715. doi:10.1016/j.cmet.2016.09.008
85. De Palma M, Biziato D, Petrova TV. Microenvironmental regulation of tumour angiogenesis. Nat Rev Cancer. 2017;17(8):457–474. doi:10.1038/nrc.2017.51
86. Yang Y, Guo Z, Chen W, et al. M2 macrophage-derived exosomes promote angiogenesis and growth of pancreatic ductal adenocarcinoma by targeting E2F2. Molecul Ther. 2021;29(3):1226–1238. doi:10.1016/j.ymthe.2020.11.024
87. Ma YS, Wu TM, Ling CC, et al. M2 macrophage-derived exosomal microRNA-155-5p promotes the immune escape of colon cancer by downregulating ZC3H12B. Mol Ther Oncolytics. 2021;20:484–498. doi:10.1016/j.omto.2021.02.005
88. Pu J, Xu Z, Nian J, et al. M2 macrophage-derived extracellular vesicles facilitate CD8+T cell exhaustion in hepatocellular carcinoma via the miR-21-5p/YOD1/YAP/β-catenin pathway. Cell Death Discovery. 2021;7(1):182. doi:10.1038/s41420-021-00556-3
89. Lu L, Ling W, Ruan Z. TAM-derived extracellular vesicles containing microRNA-29a-3p explain the deterioration of ovarian cancer. Mol Ther Nucleic Acids. 2021;25:468–482. doi:10.1016/j.omtn.2021.05.011
90. Zhou J, Li X, Wu X, et al. Exosomes released from tumor-associated macrophages transfer miRNAs that induce a treg/th17 cell imbalance in epithelial ovarian cancer. Cancer Immunol Res. 2018;6(12):1578–1592. doi:10.1158/2326-6066.Cir-17-0479
91. Binenbaum Y, Fridman E, Yaari Z, et al. Transfer of miRNA in macrophage-derived exosomes induces drug resistance in pancreatic adenocarcinoma. Cancer Res. 2018;78(18):5287–5299. doi:10.1158/0008-5472.Can-18-0124
92. Zhang F, Sang Y, Chen D, et al. M2 macrophage-derived exosomal long non-coding RNA AGAP2-AS1 enhances radiotherapy immunity in lung cancer by reducing microRNA-296 and elevating NOTCH2. Cell Death Dis. 2021;12(5):467. doi:10.1038/s41419-021-03700-0
93. Shimasaki N, Jain A, Campana D. NK cells for cancer immunotherapy. Nat Rev Drug Discov. 2020;19(3):200–218. doi:10.1038/s41573-019-0052-1
94. Myers JA, Miller JS. Exploring the NK cell platform for cancer immunotherapy. Nat Rev Clin Oncol. 2021;18(2):85–100. doi:10.1038/s41571-020-0426-7
95. Cózar B, Greppi M, Carpentier S, Narni-Mancinelli E, Chiossone L, Vivier E. Tumor-infiltrating natural killer cells. Cancer Discov. 2021;11(1):34–44. doi:10.1158/2159-8290.Cd-20-0655
96. Chiossone L, Dumas PY, Vienne M, Vivier E. Natural killer cells and other innate lymphoid cells in cancer. Nat Rev Immunol. 2018;18(11):671–688. doi:10.1038/s41577-018-0061-z
97. Jong AY, Wu CH, Li J, et al. Large-scale isolation and cytotoxicity of extracellular vesicles derived from activated human natural killer cells. J Extracell Vesicles. 2017;6(1):1294368. doi:10.1080/20013078.2017.1294368
98. Zhu L, Kalimuthu S, Gangadaran P, et al. Exosomes derived from natural killer cells exert therapeutic effect in melanoma. Theranostics. 2017;7(10):2732–2745. doi:10.7150/thno.18752
99. Di Pace AL, Tumino N, Besi F, et al. Characterization of human NK cell-derived exosomes: role of DNAM1 receptor in exosome-mediated cytotoxicity against tumor. Cancers. 2020;12(3). doi:10.3390/cancers12030661
100. Wu CH, Li J, Li L, et al. Extracellular vesicles derived from natural killer cells use multiple cytotoxic proteins and killing mechanisms to target cancer cells. J Extracell Vesicles. 2019;8(1):1588538. doi:10.1080/20013078.2019.1588538
101. Neviani P, Wise PM, Murtadha M, et al. Natural killer-derived exosomal miR-186 inhibits neuroblastoma growth and immune escape mechanisms. Cancer Res. 2019;79(6):1151–1164. doi:10.1158/0008-5472.Can-18-0779
102. Jiang Y, Jiang H, Wang K, Liu C, Man X, Fu Q. Hypoxia enhances the production and antitumor effect of exosomes derived from natural killer cells. Ann Transl Med. 2021;9(6):473. doi:10.21037/atm-21-347
103. Wculek SK, Cueto FJ, Mujal AM, Melero I, Krummel MF, Sancho D. Dendritic cells in cancer immunology and immunotherapy. Nat Rev Immunol. 2020;20(1):7–24. doi:10.1038/s41577-019-0210-z
104. Jhunjhunwala S, Hammer C, Delamarre L. Antigen presentation in cancer: insights into tumour immunogenicity and immune evasion. Nat Rev Cancer. 2021;21(5):298–312. doi:10.1038/s41568-021-00339-z
105. Matsumoto K, Morisaki T, Kuroki H, et al. Exosomes secreted from monocyte-derived dendritic cells support in vitro naive CD4+ T cell survival through NF-(kappa)B activation. Cell Immunol. 2004;231(1–2):20–29. doi:10.1016/j.cellimm.2004.11.002
106. Segura E, Nicco C, Lombard B, et al. ICAM-1 on exosomes from mature dendritic cells is critical for efficient naive T-cell priming. Blood. 2005;106(1):216–223. doi:10.1182/blood-2005-01-0220
107. Nolte-’t Hoen EN, Buschow SI, Anderton SM, Stoorvogel W, Wauben MH. Activated T cells recruit exosomes secreted by dendritic cells via LFA-1. Blood. 2009;113(9):1977–1981. doi:10.1182/blood-2008-08-174094
108. Hao S, Yuan J, Xiang J. Nonspecific CD4(+) T cells with uptake of antigen-specific dendritic cell-released exosomes stimulate antigen-specific CD8(+) CTL responses and long-term T cell memory. J Leukoc Biol. 2007;82(4):829–838. doi:10.1189/jlb.0407249
109. Chen S, Lv M, Fang S, Ye W, Gao Y, Xu Y. Poly(I:C) enhanced anti-cervical cancer immunities induced by dendritic cells-derived exosomes. International Journal of Biological Macromolecules. 2018;113:1182–1187. doi:10.1016/j.ijbiomac.2018.02.034
110. Qazi KR, Gehrmann U, Domange Jordö E, Karlsson MC, Gabrielsson S. Antigen-loaded exosomes alone induce Th1-type memory through a B-cell-dependent mechanism. Blood. 2009;113(12):2673–2683. doi:10.1182/blood-2008-04-153536
111. Näslund TI, Gehrmann U, Qazi KR, Karlsson MC, Gabrielsson S. Dendritic cell-derived exosomes need to activate both T and B cells to induce antitumor immunity. J Immunol. 2013;190(6):2712–2719. doi:10.4049/jimmunol.1203082
112. Simhadri VR, Reiners KS, Hansen HP, et al. Dendritic cells release HLA-B-associated transcript-3 positive exosomes to regulate natural killer function. PLoS One. 2008;3(10):e3377. doi:10.1371/journal.pone.0003377
113. Viaud S, Terme M, Flament C, et al. Dendritic cell-derived exosomes promote natural killer cell activation and proliferation: a role for NKG2D ligands and IL-15Ralpha. PLoS One. 2009;4(3):e4942. doi:10.1371/journal.pone.0004942
114. Munich S, Sobo-Vujanovic A, Buchser WJ, Beer-Stolz D, Vujanovic NL. Dendritic cell exosomes directly kill tumor cells and activate natural killer cells via TNF superfamily ligands. Oncoimmunology. 2012;1(7):1074–1083. doi:10.4161/onci.20897
115. Sobo-Vujanovic A, Munich S, Vujanovic NL. Dendritic-cell exosomes cross-present Toll-like receptor-ligands and activate bystander dendritic cells. Cell Immunol. 2014;289(1–2):119–127. doi:10.1016/j.cellimm.2014.03.016
116. Ruhland MK, Roberts EW, Cai E, et al. Visualizing synaptic transfer of tumor antigens among dendritic cells. Cancer Cell. 2020;37(6):786–799.e5. doi:10.1016/j.ccell.2020.05.002
117. Gabrilovich DI, Nagaraj S. Myeloid-derived suppressor cells as regulators of the immune system. Nat Rev Immunol. 2009;9(3):162–174. doi:10.1038/nri2506
118. Bronte V, Brandau S, Chen SH, et al. Recommendations for myeloid-derived suppressor cell nomenclature and characterization standards. Nat Commun. 2016;7:12150. doi:10.1038/ncomms12150
119. Fenselau C, Ostrand-Rosenberg S. Molecular cargo in myeloid-derived suppressor cells and their exosomes. Cell Immunol. 2021;359:104258. doi:10.1016/j.cellimm.2020.104258
120. Wang Y, Yin K, Tian J, et al. Granulocytic myeloid-derived suppressor cells promote the stemness of colorectal cancer cells through exosomal S100A9. Adv Sci. 2019;6(18):1901278. doi:10.1002/advs.201901278
121. Zhou JH, Yao ZX, Zheng Z, et al. G-MDSCs-derived exosomal miRNA-143-3p promotes proliferation via targeting of ITM2B in lung cancer. OncoTargets and Therapy. 2020;13:9701–9719. doi:10.2147/ott.S256378
122. Rashid MH, Borin TF, Ara R, et al. Critical immunosuppressive effect of MDSC‑derived exosomes in the tumor microenvironment. Oncol Rep. 2021;45(3):1171–1181. doi:10.3892/or.2021.7936
123. Saunderson SC, Schuberth PC, Dunn AC, et al. Induction of exosome release in primary B cells stimulated via CD40 and the IL-4 receptor. J Immunol. 2008;180(12):8146–8152. doi:10.4049/jimmunol.180.12.8146
124. Muntasell A, Berger AC, Roche PA. T cell-induced secretion of MHC class II-peptide complexes on B cell exosomes. THE EMBO Journal. 2007;26(19):4263–4272. doi:10.1038/sj.emboj.7601842
125. Klinker MW, Lizzio V, Reed TJ, Fox DA, Lundy SK. Human B cell-derived lymphoblastoid cell lines constitutively produce fas ligand and secrete MHCII(+)FasL(+) killer exosomes. Front Immunol. 2014;5:144. doi:10.3389/fimmu.2014.00144
126. Zhang F, Li R, Yang Y, et al. Specific decrease in B-cell-derived extracellular vesicles enhances post-chemotherapeutic CD8(+) T cell responses. Immunity. 2019;50(3):738–750.e7. doi:10.1016/j.immuni.2019.01.010
127. Cancer Genome Atlas Research Network. Integrated genomic analyses of ovarian carcinoma. Nature. 2011;474(7353):609–615. doi:10.1038/nature10166
128. Yang Z, Wang W, Zhao L, et al. Plasma cells shape the mesenchymal identity of ovarian cancers through transfer of exosome-derived microRNAs. Sci Adv. 2021;7(9). doi:10.1126/sciadv.abb0737
129. Komi DEA, Redegeld FA. Role of mast cells in shaping the tumor microenvironment. Clin Rev Allergy Immunol. 2020;58(3):313–325. doi:10.1007/s12016-019-08753-w
130. Skokos D, Le Panse S, Villa I, et al. Mast cell-dependent B and T lymphocyte activation is mediated by the secretion of immunologically active exosomes. J Immunol. 2001;166(2):868–876. doi:10.4049/jimmunol.166.2.868
131. Skokos D, Botros HG, Demeure C, et al. Mast cell-derived exosomes induce phenotypic and functional maturation of dendritic cells and elicit specific immune responses in vivo. J Immunol. 2003;170(6):3037–3045. doi:10.4049/jimmunol.170.6.3037
132. Li F, Wang Y, Lin L, et al. Mast cell-derived exosomes promote Th2 cell differentiation via OX40L-OX40 ligation. J Immunol Res. 2016;2016:3623898. doi:10.1155/2016/3623898
133. Xiao H, Lässer C, Shelke GV, et al. Mast cell exosomes promote lung adenocarcinoma cell proliferation – role of KIT-stem cell factor signaling. CCS. 2014;12:64. doi:10.1186/s12964-014-0064-8
134. Powell DR, Huttenlocher A. Neutrophils in the tumor microenvironment. Trends Immunol. 2016;37(1):41–52. doi:10.1016/j.it.2015.11.008
135. Giese MA, Hind LE, Huttenlocher A. Neutrophil plasticity in the tumor microenvironment. Blood. 2019;133(20):2159–2167. doi:10.1182/blood-2018-11-844548
136. Tyagi A, Wu SY, Sharma S, et al. Exosomal miR-4466 from nicotine-activated neutrophils promotes tumor cell stemness and metabolism in lung cancer metastasis. Oncogene. 2022;41(22):3079–3092. doi:10.1038/s41388-022-02322-w
137. Yang W, Zhu G, Wang S, et al. In situ dendritic cell vaccine for effective cancer immunotherapy. ACS nano. 2019;13(3):3083–3094. doi:10.1021/acsnano.8b08346
138. Ding Z, Li Q, Zhang R, et al. Personalized neoantigen pulsed dendritic cell vaccine for advanced lung cancer. Signal Transduction and Targeted Therapy. 2021;6(1):26. doi:10.1038/s41392-020-00448-5
139. Zitvogel L, Regnault A, Lozier A, et al. Eradication of established murine tumors using a novel cell-free vaccine: dendritic cell-derived exosomes. Nat Med. 1998;4(5):594–600. doi:10.1038/nm0598-594
140. Lu Z, Zuo B, Jing R, et al. Dendritic cell-derived exosomes elicit tumor regression in autochthonous hepatocellular carcinoma mouse models. J Hepatol. 2017;67(4):739–748. doi:10.1016/j.jhep.2017.05.019
141. Zhong X, Zhou Y, Cao Y, et al. Enhanced antitumor efficacy through microwave ablation combined with a dendritic cell-derived exosome vaccine in hepatocellular carcinoma. Int J Hyperth. 2020;37(1):1210–1218. doi:10.1080/02656736.2020.1836406
142. Damo M, Wilson DS, Simeoni E, Hubbell JA. TLR-3 stimulation improves anti-tumor immunity elicited by dendritic cell exosome-based vaccines in a murine model of melanoma. Sci Rep. 2015;5:17622. doi:10.1038/srep17622
143. Morse MA, Garst J, Osada T, et al. A phase I study of dexosome immunotherapy in patients with advanced non-small cell lung cancer. J Transl Med. 2005;3(1):9. doi:10.1186/1479-5876-3-9
144. Besse B, Charrier M, Lapierre V, et al. Dendritic cell-derived exosomes as maintenance immunotherapy after first line chemotherapy in NSCLC. Oncoimmunology. 2016;5(4):e1071008. doi:10.1080/2162402x.2015.1071008
145. Fan M, Liu H, Yan H, et al. A CAR T-inspiring platform based on antibody-engineered exosomes from antigen-feeding dendritic cells for precise solid tumor therapy. Biomaterials. 2022;282:121424. doi:10.1016/j.biomaterials.2022.121424
146. Phung CD, Pham TT, Nguyen HT, et al. Anti-CTLA-4 antibody-functionalized dendritic cell-derived exosomes targeting tumor-draining lymph nodes for effective induction of antitumor T-cell responses. Acta biomaterialia. 2020;115:371–382. doi:10.1016/j.actbio.2020.08.008
147. Cheng L, Wang Y, Huang L. Exosomes from M1-polarized macrophages potentiate the cancer vaccine by creating a pro-inflammatory microenvironment in the lymph node. Molecul Ther. 2017;25(7):1665–1675. doi:10.1016/j.ymthe.2017.02.007
148. Wang P, Wang H, Huang Q, et al. Exosomes from M1-polarized macrophages enhance paclitaxel antitumor activity by activating macrophages-mediated inflammation. Theranostics. 2019;9(6):1714–1727. doi:10.7150/thno.30716
149. Li J, Li N, Wang J. M1 macrophage-derived exosome-encapsulated cisplatin can enhance its anti-lung cancer effect. Minerva medica. 2020. doi:10.23736/s0026-4806.20.06564-7
150. Wang X, Ding H, Li Z, et al. Exploration and functionalization of M1-macrophage extracellular vesicles for effective accumulation in glioblastoma and strong synergistic therapeutic effects. Signal Transduction and Targeted Therapy. 2022;7(1):74. doi:10.1038/s41392-022-00894-3
151. Gunassekaran GR, Poongkavithai Vadevoo SM, Baek MC, Lee B. M1 macrophage exosomes engineered to foster M1 polarization and target the IL-4 receptor inhibit tumor growth by reprogramming tumor-associated macrophages into M1-like macrophages. Biomaterials. 2021;278:121137. doi:10.1016/j.biomaterials.2021.121137
152. Choo YW, Kang M, Kim HY, et al. M1 macrophage-derived nanovesicles potentiate the anticancer efficacy of immune checkpoint inhibitors. ACS nano. 2018;12(9):8977–8993. doi:10.1021/acsnano.8b02446
153. Wang S, Li F, Ye T, et al. Macrophage-tumor chimeric exosomes accumulate in lymph node and tumor to activate the immune response and the tumor microenvironment. Science Translational Medicine. 2021;13(615):eabb6981. doi:10.1126/scitranslmed.abb6981
154. Kaban K, Hinterleitner C, Zhou Y, et al. Therapeutic silencing of BCL-2 using NK cell-derived exosomes as a novel therapeutic approach in breast cancer. Cancers. 2021;13(10). doi:10.3390/cancers13102397
155. Han D, Wang K, Zhang T, Gao GC, Xu H. Natural killer cell-derived exosome-entrapped paclitaxel can enhance its anti-tumor effect. Eur Rev Med Pharmacol Sci. 2020;24(10):5703–5713. doi:10.26355/eurrev_202005_21362
156. Wang G, Hu W, Chen H, Shou X, Ye T, Xu Y. Cocktail strategy based on NK cell-derived exosomes and their biomimetic nanoparticles for dual tumor therapy. Cancers. 2019;11(10). doi:10.3390/cancers11101560
157. Zhang J, Ji C, Zhang H, et al. Engineered neutrophil-derived exosome-like vesicles for targeted cancer therapy. Sci Adv. 2022;8(2):eabj8207. doi:10.1126/sciadv.abj8207
158. Hong J, Kang M, Jung M, et al. T-cell-derived nanovesicles for cancer immunotherapy. Adv Mater. 2021;33(33):e2101110. doi:10.1002/adma.202101110