Analysis of the Outcomes of the Screen-Time Reduction in Computer Vision Syndrome: A Cohort Comparative Study
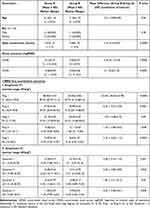
Introduction
Computer vision syndrome (CVS) is a multifactorial syndrome that basically affects the eye, the musculoskeletal system, the circadian rhythms, the behavioral and sleep patterns, the social lifestyle, the occupational performances, and the public health.1–11 The American Optometric Association defined CVS as a group of eye-related and vision-related problems that result from prolonged computer, tablet, laptop, e-reader and cell phone use.1 The ocular complaints include dry eye disease (DED), visual blur, reduced visual performances, eye strain, asthenopia, accommodation-convergence imbalance, diplopia, and near objects visualization difficulties. The extraocular complaints include sleep disturbances, insomnia, headache, depression, pain in the neck, back, and shoulders, weakness in the fingers from arthritis and tendonitis.1–34
The prevalence rate of CVS varies from less than 50% to over 90% according to age, occupation, country, and education.7–12,14–19,22,30,35–39 Unfortunately, the COVID-19 pandemic has increased the average daily students’ requirements of total screen-hours for online learning and augmented the need for the educational mandated computer system use program.9,28,30,33,35–39 Thus, we require more awareness campaigns to increase students’ awareness of CVS hazards and protective measures.9,22,29,31,34,36
Several studies concluded that the unexplained reduction in the visual functions and performances could be attributed to the smartphones and digital screens.7,8,10,13,39–47 Electronic devices or screens might be responsible for the emergence of unexplained recent era of deterioration of visual functions and performances with underlying mechanisms not fully understood.13 Reduction of the visual sensitivity in the dark with smartphone use has been reported.42 Hand-held devices are responsible for reduction of fusional convergence and recession of near point of convergence.40 Linearly polarized smartphones are responsible for asthenopia and reduced confusion flicker frequency.10 Smartphone has adverse effects on binocular vision by decreasing the binocular accommodative facility.44 Electronic devices might be responsible for accommodative disorders and convergence insufficiency in pediatric users.47 There is lack of studies in the literature investigating the macular integrity and functions.25
Recently, two studies7,8 documented the existence of screen-induced foveal dysfunction by using mfERG examination. This reduction in the foveal responses explained the associated reduction in the visual performances and considered as CVS-related visual sequelae in their conclusions.7,8 Both studies reported that the recorded mfERG findings revealed macular cone/bipolar cell dysfunction as they suggested that CVS had elicited mfERG changes from light exposure as a result of electrode-focusing effects, cone adaptation, and/or the spectral output of digital or electronic screens which varied between subjects.7,8
Therefore, we conducted this study to document the mfERG changes foveal responses in cases with screen-induced foveal dysfunction before and after strict reduction of the screen-time to ≤1 screen-hour daily as our primary outcomes. Meanwhile, our secondary outcome was discovering the associated changes in visual performances.
Methods
Our study was designed as a prospective cohort comparative study, which was approved by the Ethics Committee in the Faculty of Medicine, Sohag University, Egypt. Furthermore, the study obtained its trial registry number from the ClinicalTrials.gov (ID: NCT04405648). Our study adhered to the tenets of the Declaration of Helsinki. The nature of visual, ocular, and extraocular hazards with potential risk factors of CVS was explained carefully to all participants who signed an informed consent prior to enrolment in the study.
Our study included 49 eyes of age- and sex-matched 49 participants (students), who were divided into two groups. Group A (control group) included 25 eyes of 25 students with no CVS diagnosis while group B (CVS group) included 24 eyes of 24 students but with CVS diagnosis. In group B, all students diagnosed with CVS had fulfilled Iqbal’s criteria6–8 for CVS diagnosis, which included four major criteria. These criteria were the presence of ≥1 ocular CVS-symptoms, the presence of ≥1 extraocular CVS-symptoms, the presence of ≥1 CVS symptoms-attacks while all symptoms should be related to the time of the screen use and the presence of ≥1 CVS-signs on ophthalmic examination as DED, refractive errors, unexplained visual acuity reduction, or mfERG examination documenting screen-induced foveal dysfunction.
Our inclusion criteria in group A included normal healthy controls not diagnosed with CVS or having eye or systemic complaints, ie, no ocular or extraocular complaints. On the other hand, the inclusion criteria in group B included subjects diagnosed with CVS according to the self-assessment questionnaire and ophthalmic examination. Our exclusion criteria for both groups were concomitant eye and/or retinal pathology or infection, associated accommodation-convergence imbalance, systemic diseases, on current medication, previous eye or systemic disease or surgery and students who could not strictly reduce their-screen time.
We included only one eye for each subject in our study to reduce the potential statistical bias. At first, both eyes underwent ophthalmic and fundus examinations for each participant but only participants with both eyes fulfilling the eligibility criteria were included in this study. Thereafter, a coin toss was performed separately for each participant and only one eye (left or right eye) was randomly included for each participant. Following the coin toss, only the selected eye underwent mfERG examination.
All students were subjected to valid and reliable self-assessment CVS questionnaire-form 3 (CVS-F3)7,8 with a Cronbach’s alpha reliability coefficient of 0.742.7,8 They responded to CVS-F3 at the beginning and the end of the study four weeks. Furthermore, they were also subjected to objective complete ophthalmic examination including slit-lamp examination, visual acuity measurements of both uncorrected distance visual acuity (UDVA) and corrected distance visual acuity (CDVA) measured in logarithm of minimal angle of resolution (logMAR), manifest refraction, fundus examination and mfERG examination to document presence or absence of associated screen-induced foveal dysfunction.
Moreover, we performed the mfERG examination twice for all study participants. The first mfERG examination was performed at the time of recruiting subjects in their groups with ophthalmic examination to document any potential associated screen-induced foveal dysfunction. Meanwhile, the second mfERG examination was performed four weeks after reduction of the daily screen-hours to ≤1 hour daily to document any potential improvement in the foveal function following screen-hour reduction. Furthermore, we also recorded the outcomes of UDVA and CDVA at the time of the second mfERG.
Complete ophthalmic examinations were performed for all study participants. Our aim was to prove or exclude the CVS diagnosis according to our eligibility criteria. Meanwhile, our main interest was to focus on the mfERG examination outcomes in both groups to document potential associated screen-induced foveal dysfunction before and four weeks after screen-hour reduction.
The mfERG examination was performed using the RETIscan (Roland Instruments, Wiesbaden, Germany) according to the mfERG-standard protocol of the International Society for Clinical Electrophysiology of Vision (ISCEV). Our study protocol followed the tenets of the mfERG-standard ISCEV protocol. During mfERG examination, we used 61 hexagons mfERG-stimulus while the mfERG-normal ranges were automatically determined internally by the RETIscan device with age-matched norms. All participants were dilated prior to mfERG examination.
Nevertheless, all participants documented with complaints during ophthalmic examinations were instructed to receive the appropriate treatments. For example, participants with DED received Blink® intensive eye drops (polyethylene glycol 400 with 0.25%, sodium hyaluronate, Johnson & Johnson Vision, Irvine, California, United States) 3–5 times daily according to severity. Spectacles were prescribed for participants with refractive errors.
We faced great difficulty to convince the participants to be recruited in this study and found only limited number of students who were seriously willing to reduce their screen-time after being convinced with this idea as they wanted to experience this trial. In fact, before recruitment started; we anticipated a large number of students to participate in this study, yet unfortunately only small number of participants actually accepted to reduce their screen-time to be included in this study after they fulfilled our eligibility criteria.
Furthermore, we instructed these students to change their screen-time and style by following strict measures for four weeks to reduce their screen-time which were: specify only one hour daily to use screens according to their wishes, ie, at college or home to accomplish the necessary assignments, use only the desktop computer and/or laptop during this particular hour instead of smartphone, Ie, shift from small hand-held screens to large non-hand-held screens, stop using video-calls and use only audio-calls, temporary uninstall the social media applications from their smartphones and other screens till end of the study, study medicine using books or hard copies by printing the necessary word and/or PDF files instead of reading them from the screens; and watch the necessary teaching videos, movies and/or online lectures, meetings or courses via the TV screen from a proper distance; instead of using smartphones, laptops, pads, tabs and/or desktop computers for such purposes. Moreover, all students were requested to avoid the potential risk factors that could aggravate their CVS subjective complaints. These risk factors were mainly watching screens in the dark, close eye-screen distance with incorrect viewing distance, small font-size, poor screen-resolution and glare, texting with both thumbs, improper lightening, uncomfortable seating postures and improper gaze direction. Students, who admitted that they cannot carefully follow these instructions, were not included in this study. Meanwhile, all students were informed that they can quit anytime they want from the trial. Therefore, only 49 participants were included in this study as they managed to follow these instructions, complete the trial and fulfilled our eligibility criteria.
Statistical Analysis
Data were analyzed using STATA version 14.2 (Stata Statistical Software: Release 14.2 College Station, TX: StataCorp LP.). Quantitative data was represented as mean, standard deviation, median, and range. Student’s t-test was used to compare the mean values between two groups. When the data was not normally distributed, Mann–Whitney test was used. We compared the pre- and post-screen time reduction data either by using paired t-test if data was normally distributed and Wilcoxon matched pairs signed rank test if the data were not normally distributed. We used the Chi-squared test or Fisher’s exact test to compare between number and percentage that present the qualitative data. We used Spearman correlation test to find the correlation between different parameters. Graphs were produced by using Excel or STATA program. A P value of less than 0.05 was considered to be statistically significant.
Results
Demographic Characteristics
This study included 49 participants (students), 27 females and 23 males with mean age of 21.56±1.16 and 21.36±1.22 (mean ± standard deviation) years for both groups, respectively (P = 0.56). Table 1 shows the demographic characteristics of the studied participants in both groups. We observed no significant differences between groups A and B.
![]() |
Table 1 Comparison Between the A and B Groups Before Screen-Time Reduction |
The First mfERG Examination Outcomes
Table 1 shows the comparison between both groups regarding the mfERG outcomes at the time of first mfERG examination. We observed statistically significant differences between both groups regarding mean UDVA and mean CDVA in favor of control group (P = 0.0006 and 0.0002, respectively). Both mean UDVA and CDVA were much better in the control than the CVS group prior to screen-time reduction (Table 1).
The first mfERG outcomes revealed good foveal responses in all eyes of the control group with preserved peak in almost all mfERG 5 Rings (R1, R2, R3, R4, and R5) and 4 Quadrants (Q1, Q2, Q3 and Q4) as the first foveal peak and amplitude density (P1 AD) were mostly normal. On the contrary, the mfERG outcomes revealed great reduction in the foveal responses in almost all eyes of the CVS group in almost all mfERG Rings and Quadrants (P1 AD was below the normal age). Therefore, we exhibited statistically significant differences between both groups regarding the mean R1, R2, R5, Q1, Q2, and Q4 (P=<0.0001, 0.0001, 0.0003, 0.001, 0.002 and 0.006, respectively; Table 1).
Additionally, we observed significant differences in the mean total daily screen-hours between both groups from a mean of 2.32±1.22 screen-hours in the control to a mean of 5.48±1.92 screen-hours in the CVS group (P=<0.0001, Table 1). This means that the screen-time consumed by the CVS participants was more than double the screen-time consumed by the control participants.
The Second mfERG Examination Outcomes
Table 2 shows the outcomes of the mfERG examination before and after four weeks of screen-hour reduction to ≤1 screen-hour daily in both groups. We observed no statistically significant difference between both groups with regard to mean UDVA and CDVA (P = 0.06 and 0.09, respectively).
![]() |
Table 2 Comparison Between the A and B Groups After Screen-Time Reduction |
Furthermore, we exhibited no statistically significant differences between control and CVS group regarding the mean of all mfERG Rings and Quadrants except in mean R1, R5, Q2, and Q4 in favor of the control group (P=<0.0001, 0.0004, 0.0001 and 0.002, respectively; Table 2). Our outcomes documented greater improvements in the mean mfERG R2, R3, R4, Q1, and Q3 in the CVS group so that we observed no significant differences between them and the mean values of corresponding variables in the control group four weeks after screen-hour reduction.
Table 3 shows the outcomes of the CVS group before and four weeks after reduction of screen-hours. The mean total daily screen-hours were reduced from 5.48±1.92 to 1±0 screen-hours daily in the CVS group (P=<0.0001). At the mfERG second examination, there were significant post-reduction improvements in the mean UDVA as well as mean CDVA in the CVS group (P=<0.0001 and 0.002, respectively). Moreover, we recorded statistically significant better post-reduction improvements in the foveal responses (P1 AD close to normal) with greater improvements in the mean mfERG R1, R2, R3, R5, Q1, and Q 3 (P=<0.0001, <0.0001, 0.0005, 0.02, <0.0001 and 0.04, respectively; Table 3). On the other hand, we observed no statistically significant differences between the first and second examination outcomes in the control group regarding both visual and post-reduction mfERG outcomes.
![]() |
Table 3 Comparison of Outcomes in Group B Before and After Screen-Time Reduction |
Figure 1 shows the comparative visual and mfERG outcomes between A and B groups before and four weeks after screen-hour reduction. Figure 2 shows the mfERG outcomes in one student from the CVS group with improved foveal responses.
![]() |
Figure 2 Multifocal electroretinography showing improved foveal responses in the CVS group. (A and B) Oculus sinister of one student before and four weeks after reduction in screen-hours. |
Discussion
Our study documented the existence of the screen-induced foveal dysfunction in CVS patients using mfERG examinations. Additionally, we exhibited statistically significant post-reduction remarkable improvements in almost all mfERG Rings and Quadrants in the CVS group. Therefore, we suggest that the screen-induced foveal dysfunction might be reversible if the total screen-time reduced to ≤1 screen-hour daily. These outcomes might signify that if a CVS patient, with positive mfERG findings, managed to completely stop using screens for four weeks, his/her mfERG findings mostly will be normal.
Our screen-induced foveal dysfunction outcomes might be due to the dysfunction in the retinal bipolar cells in the macular cones. These bipolar cells are mainly the basic retinal interneurons responsible for the fast and direct visual signals pathways from the cones to the ganglion cells.48 Moreover, we think that the mfERG changes emerged from this screen-induced foveal dysfunction might originally have been elicited by the direct light exposure from the screens caused by numerous factors, such as the cone adaptation or the spectral output of the screens, which varies from device to device, and among subjects and might be related to the potential electrode/focusing effects.7,8 We posit that these interpretations might explain why the screen-induced foveal dysfunction outcomes could be reversible. Meanwhile, the longer-wavelength light high levels are more effective in adaptation of the M and L cones than the shorter-wavelength light exposure. On the other hand, we are uncertain if the study participants were using any color adjustments to their screens.
Munshi et al13 reported that CVS can be associated with unexplained visual disturbances, such as visual blur and transient loss of vision. They also reported that these unexplained visual disturbances were transient and not permanent and most of these disturbances could be alleviated by stopping the use of screens. We agree with their reports, which matches with our outcomes as we proved that the reduction in the CVS visual performances and associated visual blur was related to the screen-induced foveal dysfunction documented by the mfERG reduced foveal responses that improve greatly with reduction of the screen-time to less than one hour daily. Furthermore, our study documented statistically significant post-reduction improvements of both UDVA and CDVA associating the post-reduction improvements in the mfERG outcomes.
Recently, Iqbal et al7,8 documented the potential CVS visual sequelae. They investigated 733 and 3040 medical students, respectively, in Egyptian Universities as all students responded to the subjective self-assessment questionnaire and underwent the objective complete ophthalmic and mfERG examinations. They specified four major criteria for CVS diagnosis (Iqbal’s criteria).6–8 The first three criteria were obtained from subjective self-assessment questionnaire, which were the presence of one or more of the ocular complaints at the time of screen-use, the presence of one or more of the extraocular complaints also at the time of the screen-use, and the existence of these complain-attacks one or more times on a monthly basis over the last 12 months.6–8 The fourth criterion was objective depending on ophthalmic examination to document any of these ocular complaints and associated risk factors as DED, refractive errors, contact lens wearing, conjunctival hyperemia and/or mfERG positive findings.6–8 They revealed7,8 the existence of positive mfERG findings in high-risk CVS patients with significant reduced foveal responses associated with complaints of visual reduction and unclear details of objects. These mfERG changes were finally defined by them as screen-induced foveal dysfunction as a potential sign of CVS visual sequelae. These conclusions coincided with the outcomes of this study.
The theory of smartphone-misuse has been reported as the main cause of sudden rise in the CVS prevalence rate in the last two decades and several reports suggested that the problem is not with the smartphone itself but in the ways people handle and use the smartphone.7–10,19,39–46 Particularly, smartphone watch in the dark, close eye-screen distance with incorrect viewing distance, small font-size, poor screen-resolution and glare, texting with both thumbs, improper lightening, uncomfortable seating postures, improper gaze direction, use of old smartphone designs, prolonged screen-time and uncorrected refractive errors are the main smartphone-misuse habits and risk factors that are responsible for the development of most ocular and extraocular CVS complaints. This smartphone-misuse hypothesis coincides with our current study outcomes in one important point. We instructed all students to shift from smartphone use to desktop computer and laptop use for ≤1 screen-hour daily for complete four weeks before they underwent the second mfERG examination. We also instructed them to change their screen-style to avoid bad habits and risk factors during these four weeks of screen-time reduction. We believe that these instructions to smartphone-off with screen-shift and avoiding the associated misuse together with reduction of screen-time were mainly responsible for the great improvements in the second mfERG outcomes in the CVS group. We also believe that CVS is originally a multifactorial syndrome in which we deal with different changeable factors that vary from subject to subject and from one screen to another, yet changing the screen-style might be helpful in alleviating or minimizing the subjective complaints but the major influence in improving the objective parameters was achieved by the screen-time reduction that actually minimized the exposure time to screens thus avoiding all risk factors in one shot regardless the screen and individual variations.
Moreover, Mou et al10 revealed that smartphone with circularly polarized light was much better than smartphone with linearly polarized light in terms of alleviating CVS eye strain, DED, and ocular discomfort as circularly polarized light has closer properties of natural light.10
All students responded to CVS-F3 questionnaire at the beginning and the end of the four weeks study period reporting improvements in their subjective complaints. However, we did not statistically analyze their CVS-F3 detailed subjective outcomes as we mainly focused on analysis of the objective outcomes including UDVA, CDVA and mfERG outcomes which were the main scope of our study. Nevertheless, most participants reported improvement in many of their subjective complaints especially headache, eyestrain, visual blur and neck and shoulder pain following screen-time reduction.
The main limitations of this study were the small number of study participants (49 subjects) due to cost-issues and time-consuming nature of mfERG examinations. Moreover, the students who actually managed to strictly reduce their screen-time were few as we excluded many students who admitted they did not reduce their screen-time. Thus, finally our study included only 49 students who achieved our eligibility criteria.
In conclusion, the current study outcomes proved the existence of screen-induced foveal dysfunction as mfERG sign of CVS. We think that the mfERG changes elicited by the screen-induced foveal dysfunction are reversible with strict reduction of screen-time to ≤1 screen-hour daily resulting in significant post-reduction improvements in the foveal responses. Additionally, the great significant post-reduction improvements in both mean UDVA and CDVA that associated the foveal responses improvements support our conclusion of the reversibility of the adverse effects of screen-induced foveal dysfunction with strict screen-time reduction. Therefore, we posit that the complete stoppage of using screens, if possible, is capable of regaining the original foveal functions and visual performances. Furthermore, future studies with larger sample size are recommended.
Data Sharing Statement
Data are available upon reasonable request to the authors.
Acknowledgments
We are grateful to Dr. Mona AboAli, Mr. Hamza Mohammed, Seif Mohammed, Lina Mohammed as well as the EPK Group for their great support throughout this study. The authors are also grateful for the great help and support of Professor Fouad Metry, the mathematician expert who analyzed the study statistics.
Disclosure
The authors report no conflicts of interest in this work.
References
1. American Optometric Association. Computer vision syndrome. Available from: https://www.aoa.org/patients-and-public/caring-for-your-vision/protecting-your-vision/computer-vision-syndrome. Accessed June 23, 2018.
2. Vaz FT, Henriques SP, Silva DS, Roque J, Lopes AS, Mota M. Digital asthenopia: Portuguese Group of ergophthalmology survey. Acta Med Port. 2019;32(4):260–265. doi:10.20344/amp.10942
3. Ahmed SF, McDermott KC, Burge WK, et al. Visual function, digital behavior and the vision performance index. Clin Ophthalmol. 2018;12:2553–2561. doi:10.2147/OPTH.S187131
4. Lee YK, Chang CT, Lin Y, Cheng ZH. The dark side of smartphone usage: psychological traits, compulsive behavior and technostress. Comput Human Behav. 2014;31:373–383. doi:10.1016/j.chb.2013.10.047
5. Touitou Y, Touitou D, Reinberg A. Disruption of adolescents’ circadian clock: the vicious circle of media use, exposure to light at night, sleep loss and risk behaviors. J Physiol Paris. 2016;110:467–479. doi:10.1016/j.jphysparis.2017.05.001
6. Iqbal M, Ibrahim Elzembely H, Said OM. Letter to the editor: “self-reported student awareness and prevalence of computer vision syndrome during COVID-19 pandemic at Al-Baha University” [Letter]. Clin Optom. 2022;14:193–194. doi:10.2147/OPTO.S391171
7. Iqbal M, Said O, Ibrahim O, Soliman A. Visual sequelae of computer vision syndrome: a cross-sectional case-control study. J Ophthalmol. 2021;2021:6630286. doi:10.1155/2021/6630286
8. Iqbal M, Elzembely H, El-Massry A. Computer vision syndrome prevalence and ocular sequelae among medical students: a university-wide study on a marginalized visual security issue. Open Ophthalmol J. 2021;15:156–170. doi:10.2174/1874364102115010156
9. Roy S, Sharif AB, Chowdhury S, et al. Unavoidable online education due to COVID-19 and its association to computer vision syndrome: a cross-sectional survey. BMJ Open Ophthalmol. 2022;7:e001118. doi:10.1136/bmjophth-2022-001118
10. Mou Y, Shen X, Yuan K, et al. Comparison of the influence of light between circularly polarized and linearly polarized smartphones on dry eye symptoms and asthenopia. Clin Transl Sci. 2022;15(4):994–1002. doi:10.1111/cts.13218
11. Cartes C, Segovia C, Salinas-Toro D, et al. Dry eye and visual display terminal-related symptoms among university students during the coronavirus disease pandemic. Ophthalmic Epidemiol. 2022;29(3):245–251. doi:10.1080/09286586.2021.1943457
12. Das A, Shah S, Adhikari TB, et al. Computer vision syndrome, musculoskeletal, and stress-related problems among visual display terminal users in Nepal. PLoS One. 2022;17(7):e0268356. doi:10.1371/journal.pone.0268356
13. Munshi S, Varghese A, Dhar-Munshi S. Computer vision syndrome-A common cause of unexplained visual symptoms in the modern era. Int J Clin Pract. 2017;71(7):e12962. doi:10.1111/ijcp.12962
14. Zalat MM, Amer SM, Wassif GA, El Tarhouny SA, Mansour TM. Computer vision syndrome, visual ergonomics and amelioration among staff members in a Saudi medical college. Int J Occup Saf Ergon. 2022;28(2):1033–1041. doi:10.1080/10803548.2021
15. Fernandez-Villacorta D, Soriano-Moreno AN, Galvez-Olortegui T, Agui-Santivañez N, Soriano-Moreno DR, Benites-Zapata VA. Computer visual syndrome in graduate students of a private university in Lima, Perú. Arch Soc Esp Oftalmol. 2021;96(10):515–520. doi:10.1016/j.oftale.2020.12.009
16. AlDarrab A, Khojah AA, Al-Ghazwi MH, et al. Magnitude and determinants of computer vision syndrome among college students at a Saudi university. Middle East Afr J Ophthalmol. 2022;28(4):252–256. doi:10.4103/meajo.meajo_272_21
17. Coronel-Ocampos J, Gómez J, Gómez A, Quiroga-Castañeda PP, Valladares-Garrido MJ. Computer visual syndrome in medical students from a private university in Paraguay: a survey study. Front Public Health. 2022;10:935405. doi:10.3389/fpubh.2022.935405
18. Alhasan AS, Aalam WA. Magnitude and determinants of computer vision syndrome among radiologists in Saudi Arabia: a national survey. Acad Radiol. 2022;29(9):e197–e204. doi:10.1016/j.acra.2021.10.023
19. AlDarrab A. Awareness and practice regarding use of digital devices and ocular health among Saudi adolescents. Oman J Ophthalmol. 2022;15(1):73–77. doi:10.4103/ojo.ojo_283_21
20. Stringham J, Stringham N, O’Brien K. Macular carotenoid supplementation improves visual performance, sleep quality, and adverse physical symptoms in those with high screen time exposure. Foods. 2017;6(7). doi:10.3390/foods6070047
21. Chawla A, Lim TC, Shikhare SN, Munk PL, Peh WCG. Computer vision syndrome: darkness under the shadow of light. Can Assoc Radiol J. 2019;70(1):5–9. doi:10.1016/j.carj.2018.10.005
22. Abuallut I, Ajeebi RE, Bahari AY, et al. Prevalence of computer vision syndrome among school-age children during the COVID-19 pandemic, Saudi Arabia: a cross-sectional survey. Children. 2022;9(11):1718. doi:10.3390/children9111718
23. Vilela M, Pellanda L, Cesa C, Castagno V. Asthenopia prevalence and risk factors associated with professional computer use-a systematic review. Int J Adv Med Sci. 2015;3(2):51–60.
24. Parihar JK, Jain VK, Chaturvedi P, Kaushik J, Jain G, Parihar AK. Computer and visual display terminals (VDT) vision syndrome (CVDTS). Med J Armed Forces India. 2016;72(3):270–276. doi:10.1016/j.mjafi.2016.03.016
25. Lawrenson JG, Hull CC, Downie LE. The effect of blue-light blocking spectacle lenses on visual performance, macular health and the sleep-wake cycle: a systematic review of the literature. Ophthalmic Physiol Opt. 2017;37(6):644–654. doi:10.1111/opo.12406
26. Billones RK, Bedruz RA, Arcega ML, et al. Digital eye strain and fatigue recognition using electrooculogram signals and ultrasonic distance measurements. In: 2018 IEEE 10th International Conference on Humanoid, Nanotechnology, Information Technology, Communication and Control, Environment and Management (HNICEM), held on November 29, 2018. Piscataway, NJ: IEEE; 2018:1–6. doi: 10.1109/HNICEM.2018.8666298.
27. Dhar-Munshi S, Amed S, Munshi S. Computer vision syndrome: an update. Br J Neurosci Nurs. 2019;15(Sup2):S10. doi:10.12968/bjnn.2019.15.Sup2.S10
28. Alatawi SK, Allinjawi K, Alzahrani K, Hussien NK, Bashir M, Ramadan EN. Self-reported student awareness and prevalence of computer vision syndrome during COVID-19 pandemic at Al-Baha university. Clin Optom. 2022;14:159–172. doi:10.2147/OPTO.S374837
29. Kim DJ, Lim C-Y, Gu N, Park CY. Visual fatigue induced by viewing a tablet computer with a high-resolution display. Korean J Ophthalmol. 2017;31(5):388–393. doi:10.3341/kjo.2016.0095
30. Wangsan K, Upaphong P, Assavanopakun P, et al. Self-reported computer vision syndrome among Thai university students in virtual classrooms during the COVID-19 pandemic: prevalence and associated factors. Int J Environ Res Public Health. 2022;19(7):3996. doi:10.3390/ijerph19073996
31. Akkaya S, Atakan T, Acikalin B, Aksoy S, Ozkurt Y. Effects of long-term computer use on eye dryness. North Clin Istanb. 2018;5(4):319–322. doi:10.14744/nci.2017.54036
32. Experience Life. Are smartphones causing thumb tendonitis? Available from: https://experiencelife.com/article/smartphones-thumb-tendonitis. Accessed June 23, 2019.
33. Li L, Zhang J, Chen M, et al. Contribution of total screen/online-course time to asthenopia in children during COVID-19 pandemic via influencing psychological stress. Front Public Health. 2021;9:736617. doi:10.3389/fpubh.2021.736617
34. Sheppard AL, Wolffsohn JS. Digital eye strain: prevalence, measurement and amelioration. BMJ Open Ophthalmol. 2018;3(1):e000146. doi:10.1136/bmjophth-2018-000146
35. Dossari SK, AlZahrani R, Alutaibi H, et al. The effect of online education on healthy eyes of Saudi teachers in the COVID-19 pandemic: a local study. Cureus. 2022;14(5):e24721. doi:10.7759/cureus.24721
36. Li R, Ying B, Qian Y, et al. Prevalence of self-reported symptoms of computer vision syndrome and associated risk factors among school students in China during the COVID-19 pandemic. Ophthalmic Epidemiol. 2022;29(4):363–373. doi:10.1080/09286586.2021.1963786
37. Artime-Ríos E, Suárez-Sánchez A, Sánchez-Lasheras F, Seguí-Crespo M. Computer vision syndrome in healthcare workers using video display terminals: an exploration of the risk factors. J Adv Nurs. 2022;78(7):2095–2110. doi:10.1111/jan.15140
38. Akiki M, Obeid S, Salameh P, et al. Association between computer vision syndrome, insomnia, and migraine among Lebanese adults: the mediating effect of stress. Prim Care Companion CNS Disord. 2022;24(4):21m03083. doi:10.4088/PCC.21m03083
39. Seresirikachorn K, Thiamthat W, Sriyuttagrai W, et al. Effects of digital devices and online learning on computer vision syndrome in students during the COVID-19 era: an online questionnaire study. BMJ Paediatr Open. 2022;6(1):e001429. doi:10.1136/bmjpo-2022-001429
40. Jaiswal S, Asper L, Long J, Lee A, Harrison K, Golebiowski B. Ocular and visual discomfort associated with smartphones, tablets and computers: what we do and do not know. Clin Exp Optom. 2019;102(5):463–477. doi:10.1111/cxo.12851
41. Long J, Cheung R, Duong S, Paynter R, Asper L. Viewing distance and eyestrain symptoms with prolonged viewing of smartphones. Clin Exp Optom. 2017;100(2):133–137. doi:10.1111/cxo.12453
42. Alim-Marvasti A, Bi W, Mahroo OA, Barbur JL, Plant GT. Transient smartphone “blindness”. N Engl J Med. 2016;374(25):2502–2504. doi:10.1056/NEJMc1514294
43. Moon JH, Kim KW, Moon NJ. Smartphone use is a risk factor for pediatric dry eye disease according to region and age: a case control study. BMC Ophthalmol. 2016;16(1):188. doi:10.1186/s12886-016-0364-4
44. Golebiowski B, Long J, Harrison K, Lee A, Chidi-Egboka N, Asper L. Smartphone use and effects on tear film, blinking and binocular vision. Curr Eye Res. 2020;45(4):428–434. doi:10.1080/02713683.2019
45. Kim J, Hwang Y, Kang S, et al. Association between exposure to smartphones and ocular health in adolescents. Ophthalmic Epidemiol. 2016;23(4):269–276. doi:10.3109/09286586.2015.1136652
46. Hue JE, Rosenfield M, Saá G. Reading from electronic devices versus hardcopy text. Work. 2014;47(3):303–307. doi:10.3233/WOR-131777
47. Pavel IA, Savu B, Chiriac CP, Bogdănici CM. Ocular and musculoskeletal changes in the pediatric population using gadgets. Rom J Ophthalmol. 2022;66(3):257–264. doi:10.22336/rjo.2022.48
48. Kaneko A. Retinal bipolar cells: their function and morphology. Trends Nuerosci. 1983;6(1):219–223. doi:10.1016/0166-2236(83)90098-X