Visualizing a complex electron wavefunction using high-resolution attosecond technology
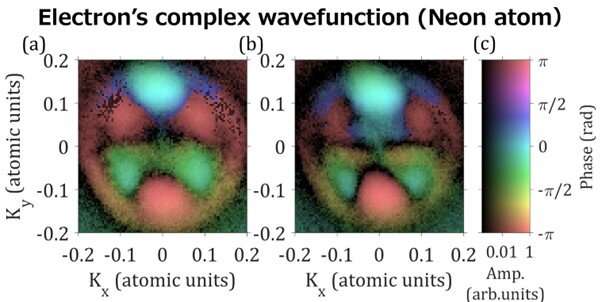
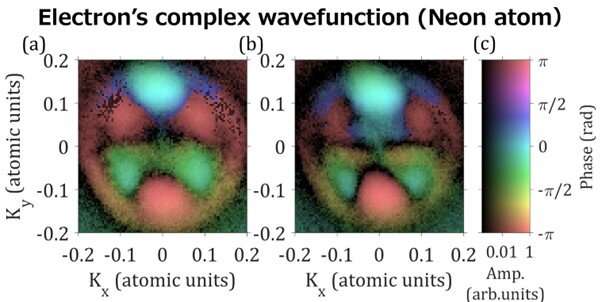
The early 20th century saw the advent of quantum mechanics to describe the properties of small particles, such as electrons or atoms. Schrödinger’s equation in quantum mechanics can successfully predict the electronic structure of atoms or molecules. However, the “duality” of matter, referring to the dual “particle” and “wave” nature of electrons, remained a controversial issue. Physicists use a complex wavefunction to represent the wave nature of an electron.
“Complex” numbers are those that have both “real” and “imaginary” parts—the ratio of which is referred to as the “phase.” However, all directly measurable quantities must be “real”. This leads to the following challenge: when the electron hits a detector, the “complex” phase information of the wavefunction disappears, leaving only the square of the amplitude of the wavefunction (a “real” value) to be recorded. This means that electrons are detected only as particles, which makes it difficult to explain their dual properties in atoms.
The ensuing century witnessed a new, evolving era of physics, namely, attosecond physics. The attosecond is a very short time scale, a billionth of a billionth of a second. “Attosecond physics opens a way to measure the phase of electrons. Achieving attosecond time-resolution, electron dynamics can be observed while freezing molecular motion,” explains Professor Hiromichi Niikura from the Department of Applied Physics, Waseda University, Japan, who, along with Professor D. M. Villeneuve—a principal research scientist at the Joint Attosecond Science Laboratory, National Research Council, and adjunct professor at University of Ottawa—pioneered the field of attosecond physics.
Niikura and Villeneuve had previously developed a breakthrough method, attosecond re-collision, and also demonstrated the imaging of a molecular orbital or electron wavefunction in a molecule.
In a recent study published in Physical Review A, these researchers employed another approach involving attosecond physics, using an attosecond laser pulse, or high-harmonic generation, to visualize a complex wavefunction. The attosecond laser pulse consists of coherent light with a wavelength much shorter than ultra-violet, referred to as extreme ultra-violet (EUV) light. When this pulse irradiates a gas, an electron is ejected.
This process is referred to as photoionization. The attosecond pulse consists of a set of “harmonics” or different colors of light. By controlling the generation of the attosecond pulse, the researchers isolated two photoionization pathways—one consisting of a particular harmonic, and the other consisting of another harmonic along with an infrared pulse—to ionize neon. The electron wavefunctions produced by both pathways can interfere with each other.
The interference pattern varies with the attosecond delay between the harmonics and the IR pulses. The team determined the phase and amplitude distributions of the photoelectron from the interference pattern and visualized its complex wavefunction. As the energy resolution is smaller than the bandwidth of the attosecond pulses, the researchers were successful in visualizing the detailed wavefunction structure.
Furthermore, the researchers developed a method of disentangling the measured wavefunction into wavefunctions that are produced by individual ionization pathways.
Now that the researchers have successfully visualized the complex wavefunction of an electron—something that cannot be seen through conventional photoelectron spectroscopy—there’s so much more they can achieve. Niikura says, “Nowadays, photoelectron spectroscopy using EUV and X-ray has become a basic tool for investigating structures and dynamics of materials. The present method will provide a way to elucidate the quantum properties of electrons.”
Visualizing the complete, detailed, complex electron wavefunction will be of significant impact in the fields of nanotechnology, chemistry, and molecular biology.
More information:
Takashi Nakajima et al, High-resolution attosecond imaging of an atomic electron wave function in momentum space, Physical Review A (2022). DOI: 10.1103/PhysRevA.106.063513
Andrew S. Maxwell et al, Entanglement of orbital angular momentum in non-sequential double ionization, Nature Communications (2022). DOI: 10.1038/s41467-022-32128-z
J. Itatani et al, Tomographic imaging of molecular orbitals, Nature (2004). DOI: 10.1038/nature03183
Provided by
Waseda University
Citation:
Visualizing a complex electron wavefunction using high-resolution attosecond technology (2023, January 11)
retrieved 11 January 2023
from https://phys.org/news/2023-01-visualizing-complex-electron-wavefunction-high-resolution.html
This document is subject to copyright. Apart from any fair dealing for the purpose of private study or research, no
part may be reproduced without the written permission. The content is provided for information purposes only.