New discovery paves the way for finding the holy grail of quantum computing
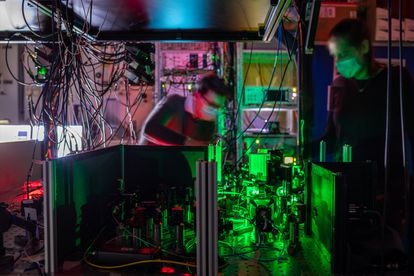
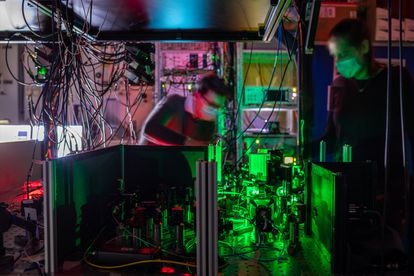
Accidental discoveries happen all the time in science and technology. Some of the best known examples include the microwave oven, penicillin, Teflon, vulcanized rubber and Viagra. It happened again at the Spanish National Research Council’s (CSIC) Materials Science Institute (ICMM) in Madrid. Elsa Prada, Ramón Aguado and Pablo San José comprised the ICMM team that collaborated with the Institute of Science and Technology of Austria (ISTA), the Catalán Institute of Nanoscience and Nanotechnology (ICN2) in Barcelona, and Princeton University in the United States. They were all working on a quest for the holy grail of quantum physics: the Majorana particle. Hypothesized by Ettore Majorana in 1937, this element of particle physics has remained in the realm of theory for 86 years. Proving the existence of the Majorana particle, also called a fermion, requires exceptional stability that can only be provided by a special material known as a topological superconductor. After two years, the international research team thought they had found the elusive particle, but further analysis revealed it to be a mirage. What they actually discovered was something equally as important – an impostor particle that mimics the behavior of a Majorana particle.
The discovery, recently published in Nature, is significant for a number of reasons. Not only does it provide a deeper understanding of topological superconductors, it demonstrates techniques for distinguishing impostor particles from real Majorana particles. It also identifies a source of error in the interpretation of experiments and points the way to an even more transcendental finding that, according to physicist Ramón Aguado, “will be a Nobel Prize winner when it can be irrefutably demonstrated because its attributes will be so superior to the standard model of fermions and bosons.”
Quantum computing exploits a unique property called superposition to exponentially increase processing capacity. While the classic bit can only have one of two values (0 or 1), a qubit (the quantum analog) is able to express several states simultaneously. But superposition requires an as-yet unachieved coherence of quantum states that must be maintained for a minimum time period. Any environmental disturbance (temperature, vibration, remanent energy, electromagnetic radiation or other common phenomena) negates the property, and causes decoherence and faults that curtail computational capacity.
Jian-Wei Pan, China’s top computer expert, summed it up neatly. “Building a practical and fault-tolerant quantum computer is one of our greatest challenges. The most formidable obstacle to building a large-scale, universal quantum computer is the presence of noise and imperfections.”
The current approach is to recognize and address these limitations of quantum computing by correcting errors using traditional processing. Another approach is to isolate quantum computers from the environment as much as possible and maintain them in temperatures close to absolute zero (-273.15°C or -459.67°F).
“A quantum computer,” explained Pablo San José, “must be completely isolated from the environment while it is working. There can be no light penetration, vibrations or any disturbance from the outside world. It has to be in a bubble, like a mini-universe unto itself. That makes it incredibly fragile.”
A quantum computer has to be in a bubble, like a mini-universe unto itself. That makes it incredibly fragile
Pablo San José, physicist
To make the great leap forward in quantum computing, the Majorana particle must be found and mastered because this particle “is able to hide the quantum information it encodes, making it invisible to the outside world,” said San José in an admittedly oversimplified explanation. “A qubit based on Majorana states would be much more robust against decoherence, since it is constructed from spatially separated quantum wave functions that make it immune to any local disturbance. This robustness would greatly facilitate the problem of scalability [creating computers with more qubits to exceed traditional computing capacity],” said Aguado. “We have been looking for this famous particle in topological superconductors for 10 or 12 years now.”
Elsa Prada says that finding the particle requires development of topological superconductors that can truly hide quantum information to protect it from external disturbances. “This type of material does not naturally exist – it is the product of materials engineering. Unfortunately, they harbor all kinds of impostor particles that can be misleading. To prevent deception by impostor particles, two things are needed: substantial improvements in the quality of the materials (a very delicate process that only a handful of materials producers know how to do), and subjecting the topological superconductor to very sophisticated measurement protocols that reveal quantum entanglement.”
A team led by Charles Marcus at the Niels Bohr Institute in Denmark took the first step by using a proprietary topological material and an innovative technique to identify the Majorana particle. The measurements pointed to an apparently correct path. The Austrian team replicated the experiment independently using the same material, and the results initially matched. But two tests are insufficient in the world of science and technology, so a complementary test was conducted. “They identified a contradiction in the conclusions, and it was an irreconcilable paradox that they couldn’t explain,” said San José. But the ICMM team found the answer – it was an impostor particle that behaved like a Majorana.
“Impostor particles often have some of the properties of real Majoranas, such as zero energy, zero spin and zero charge. But they lack the fundamental property of shielding quantum information from the environment by means of a quantum wave function that is analogous to an electron split into two spatially separated halves. In that sense, impostor particles are useless in quantum computing,” said San José.
What initially seemed like bad news, says Prada, was nevertheless a significant finding. In the complicated game of quantum Clue, the ICMM team located the impostor, which enabled them to identify the cause of errors in previous experiments – a producer of false positives. The discovery also lights the way to the development of more robust topological superconductors. “Making superconductors topological is really very complicated. You have to mix various materials very precisely, with very specific geometries, subject them to external fields, and more,” said Prada.
This scientific setback will actually help build momentum in the field. “We are too impatient,” said Aguado. “The materials Elsa Prada was talking about are 13 years old, and everyone rushed to demonstrate them. To provide some context, transistors were discovered in the 1940s. but we didn’t have mass-consumption microelectronics until the 1980s. The first microprocessors were very bulky and had around 1,000 transistors. Today, microprocessors hold more than 100 billion transistors that are only slightly larger than a few atoms of silicon… The first quantum bit based on superconducting circuits was demonstrated in 1998, and it took more than 20 years for Google and IBM to launch quantum computers with more than 10 qubits. We are just beginning to explore some very new physics concepts that will eventually lead to the next step – the Majorana-based topological qubit.”
Thus, in quantum physics, detecting false shortcuts is equally important to finding the way forward. “We are entering a barely explored technological universe. Manipulating the quantum world is a much more complicated and delicate game,” said San José. “We lack the tools and materials needed to open the door all the way. But these initial steps are crucial. In the long run, topological materials will spark a revolution that goes far beyond the quantum computer. We are facing a new frontier in the understanding of matter.”
The ICMM team said: “It’s very important to understand the fundamental physics that governs these superconducting devices. Our work enables the identification of false positives in the search for the elusive Majorana particle. When it’s finally found, we will be able to exploit the full power of quantum computing.”
Sign up for our weekly newsletter to get more English-language news coverage from EL PAÍS USA Edition