Feb 01, 2023 |
|
(Nanowerk Spotlight) Spintronics is a technology that utilizes the spin of electrons – in addition to their charge – in order to store and process information. Unlike traditional electronics, which rely on the movement of electrons to perform their functions, spintronics uses the intrinsic angular momentum of electrons to achieve the same results.
|
Contents
|
|
What is spintronics?
|
The term spintronics originally was an acronym for SPIN TRansport electrONICS. Nowadays this term generally stands for ‘spin-based electronics’ and is more broadly used for all kinds of spin polarized transport phenomena in metals, semiconductors, and oxide materials.
|
Every electron has a certain mass, a certain charge and a certain magnetic moment, or as physicists call it, a spin. The electron is not physically spinning around, but it has a magnetic north pole and a magnetic south pole. Its spin depends on which pole is pointing up. The spin of an electron can be either ‘up’ or ‘down’, represented by binary values of 1 or 0.
|
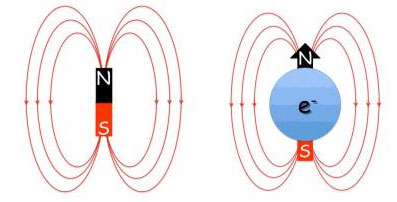 |
Just like a magnet with a north and a south pole (left), electrons are surrounded by a magnetic field (right). This magnetic momentum, or spin, could be used to store information in more efficient ways. (Illustration: Philippe Jacquod)
|
Spintronics offers the potential to address some limitations of traditional, charge-based computing. In conventional microprocessors, information is only retained when the device is powered on, which results in a delay in startup and loss of data in working memory if power is lost.
|
Additionally, charge-based microprocessors are not energy efficient because they require a constant current to maintain the data in working memory. In contrast, spintronics utilizes the magnetic properties of electrons to store information, allowing data to be retained even when the device is powered off, which can greatly reduce energy consumption. The field also has the potential for developing new types of devices such as spin-based transistors and logic gates.
|
How Spintronics Works
|
The manipulation and control of the spin of electrons is achieved through the use of specialized materials and devices such as magnetic tunnel junctions and spin valves, which can manipulate and detect the electron spin state. Specialized materials that allow for the manipulation and control of the spin of electrons include ferromagnetic metals such as iron, cobalt, and nickel, as well as Heusler alloys, diluted magnetic semiconductors (DMS) like GaMnAs, half-metallic materials such as CrO2, graphene, and topological insulators.
|
Spintronics devices can be divided into two categories: spin-transfer torque (STT) devices and spin-polarized tunneling (SPT) devices. STT devices utilize the transfer of spin angular momentum from a spin-polarized current to a magnetic layer to manipulate its magnetization, while SPT devices use quantum mechanical tunneling of electrons to detect magnetic fields and store information.
|
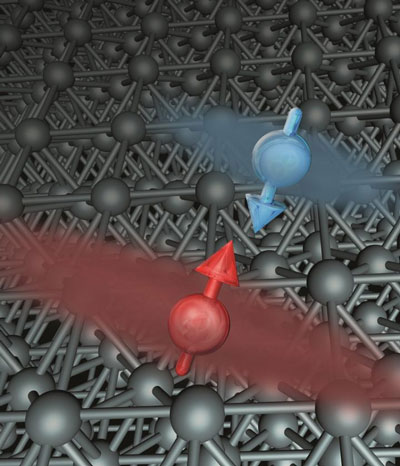 |
This is a diagram illustrating spin-up (red) and spin-down (blue) electrons in a crystal grid. (Illustration: Condensed Matter Theory Group, JGU)
|
History of Spintronics
|
The origin of spintronics encompasses a range of prior and contemporaneous discoveries and developments in the field of solid-state physics and electronics. The field of spintronics can be traced back to the 1970s, when tunneling measurements on junctions between very thin superconducting aluminum films and ferromagnetic nickel films in a high magnetic field by Robert Meservey and Peter Tedrow showed that the tunneling current was spin dependent. These experiments marked the first efforts to study spin-dependent electron transport phenomena.
|
In 1975, Michel Julliere added to this foundation by conducting initial experiments on magnetic tunnel junctions, which involved the study of spin-polarized electrons tunneling through a thin insulating barrier. Another important milestone was the observation of spin-polarized electron injection from a ferromagnetic metal to a normal metal by Johnson and Silsbee in 1985. These early experiments laid the foundation for the development of spintronics.
|
In 1988, Albert Fert of France and Peter Grünberg of Germany independently discovered Giant Magnetoresistance, a new physical effect. They both shared the 2007 Nobel Prize in Physiscs for their discovery, which further advanced the field by demonstrating the importance of spin-dependent transport in the resistance of magnetic systems.
|
In a GMR system, minor magnetic changes result in significant changes in electrical resistance. This makes GMR an effective tool for converting magnetically stored information into electrical current in hard disk drives. As a result, researchers and engineers soon started developing GMR-based read-out heads, leading to the launch of the first GMR-based read-out head in 1997, which quickly became the industry standard. Today’s advanced read-out technologies are still based on the GMR effect.
|
A hard disk stores information, such as music, in the form of microscopic areas magnetized in different directions. The information is retrieved by a read-out head that scans the disk and detects the magnetic changes. As hard disk drives become smaller and more compact, the magnetic areas also become smaller and weaker, requiring more sensitive read-out heads to retrieve the information. GMR-based read-out heads are ideal for this purpose because they can convert even the smallest magnetic changes into electrical resistance changes, which are then translated into current changes emitted by the read-out head. These current changes represent binary code, such as ones and zeros.
|
GMR became possible due to advancements in the production of ultra-thin layers of various materials during the 1970s. To work effectively, GMR requires the creation of multi-layer structures with layers only a few atoms thick. This makes GMR one of the early real-world applications of nanotechnology.
|
In the 1990s, research in the field expanded to include other spin-based phenomena such as tunnel magnetoresistance (TMR) and the manipulation of the spin of individual electrons using the technique of spin injection.
|
Tunnel magnetoresistance (TMR) is a magnetic effect observed in magnetic tunnel junctions (MTJs) consisting of two ferromagnetic layers separated by a thin insulating layer. If the insulating layer is thin enough (a few nanometers), electrons can tunnel from one ferromagnetic layer to the other, leading to TMR which is a result of the quantum mechanical properties of electrons, specifically their spin.
|
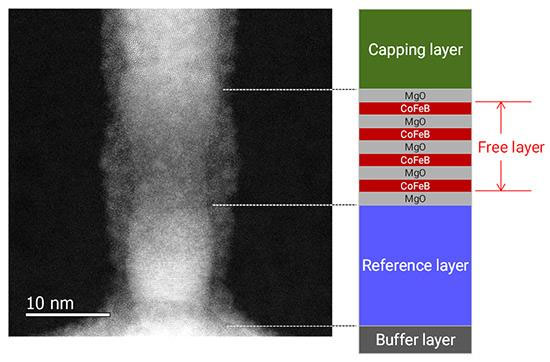 |
Transmission electron microscope image and film stack of a MTJ with a multilayered ferromagnetic structure that allows fast switching down to 3.5 ns. Interfacial anisotropy is enhanced by increasing the number of CoFeB/MgO layers. (Image: Butsurin Jinnai and Shunsuke Fukami)
|
Spin injection is a crucial process in spintronics, transferring spin-polarized electrons from a ferromagnetic electrode into a semiconductor material. The transfer of spin polarization is important for manipulating and controlling the spin of electrons in electronic devices, and is crucial for the development of spin-based technologies, such as MRAM and spin-FETs. Spin injection can be achieved through electrical or optical techniques. In 2007, researchers demonstrated efficient electrical spin injection from a ferromagnetic metal contact into silicon, producing a large electron spin polarization in the silicon.
|
The process involves the transfer of spin angular momentum from one material to another through a non-magnetic conductor, such as a metal or semiconductor. This transfer of spin polarization is important in spintronics as it allows for the manipulation and control of the spin of electrons in electronic devices. Spin injection can be achieved through various methods, including electrical and optical techniques, and is crucial for the development of spin-based technologies such as magnetic random-access memory (MRAM) and spin-field-effect transistors (spin-FETs).
|
These developments led to the creation of new types of devices such as spin valves and magnetic tunnel junctions, which are now widely used in data storage applications such as hard disk drives.
|
In recent years, research in spintronics has focused on the development of new materials and devices that can be used in a variety of applications beyond data storage, including high-speed computing, energy-efficient electronics, and quantum computing. There is also ongoing research on how to manipulate the spin states of electrons at the atomic scale using techniques such as scanning tunneling microscopy, which could lead to the creation of even more advanced and powerful spintronic devices in the future.
|
Spintronics and 2D Materials
|
Two-dimensional (2D) materials have become an active area of research in the field of spintronics because of their unique electronic, optical, and magnetic properties. The thinness and planar geometry of 2D materials give them several advantages over traditional 3D materials, making them ideal for use in spintronics applications.
|
One of the key advantages of 2D materials in spintronics is their high surface-to-volume ratio, which makes it easier to access and manipulate the magnetic moments on their surfaces. This is particularly important in applications such as magnetic memory devices, where the ability to read and write information depends on the interaction between the magnetic moments and magnetic read heads.
|
Another advantage of 2D materials in spintronics is their low dimensionality, which can lead to enhanced spin-orbit coupling, spin-spin interactions, and magnetic anisotropy. These properties can be used to control and manipulate the spins of electrons, which is essential for many spintronics applications.
|
In addition to these intrinsic properties, 2D materials can also be combined with other materials to form heterostructures that have unique and tunable magnetic properties. For example, stacking different 2D materials on top of each other can lead to the formation of hybrid structures with enhanced magnetic properties, making them suitable for use in spintronics devices.
|
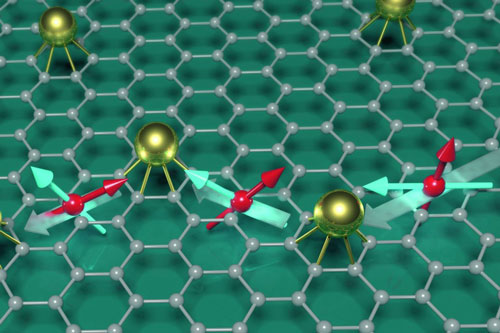 |
Illustration of electron spin in a graphene lattice. (Image: Bart van Wees)
|
Graphene in particular is a promising material for spintronics applications owing to its capacity for room-temperature spin transport over relatively long diffusion lengths of several micrometres. Graphene also has high electron mobility, and a tuneable charge carrier concentration. Also, graphene layered with magnetic materials could drive ultrathin spintronics.
|
Overall, the combination of unique electronic, optical, and magnetic properties, as well as the ability to form heterostructures, makes 2D materials a promising platform for developing new and advanced spintronics devices.
|
In 2D magnetic materials, the spin ordering is an important factor that determines the magnetic properties of the material, such as its magnetic anisotropy and its response to external magnetic fields. Understanding spin ordering in 2D magnetic materials is important for developing new technologies such as spintronics and quantum computing, which rely on the manipulation of magnetic moments to store and process information.
|
Spin ordering in 2D magnetic materials refers to the arrangement of magnetic moments (spins) in a two-dimensional material. Magnetic moments can be thought of as tiny bar magnets that interact with each other, and the direction of their spins determines their magnetic state.
|
In 2D magnetic materials, the magnetic moments are arranged in a particular pattern that gives the material its magnetic properties. There are several types of spin ordering in 2D magnetic materials, including ferromagnetic ordering, antiferromagnetic ordering, and ferrimagnetic ordering.
|
Ferromagnetic ordering is a type of spin ordering where the magnetic moments are aligned in the same direction, leading to a net magnetic moment in the material. This type of ordering is commonly found in metals such as iron, nickel, and cobalt.
|
Antiferromagnetic ordering is a type of spin ordering where the magnetic moments are aligned in opposite directions, resulting in a net magnetic moment of zero. This type of ordering is found in materials such as antiferromagnetic insulators and some layered transition metal compounds.
|
Ferrimagnetic ordering is a type of spin ordering where the magnetic moments are aligned in opposite directions, but not perfectly so. This results in a net magnetic moment that is smaller than the magnetic moments of the individual spins. Ferrimagnetic ordering is found in materials such as magnetic oxides and some layered transition metal compounds.
|
More recently, by using photons and electron spin qubits to control nuclear spins in a two-dimensional material, researchers have opened a new frontier in quantum science and technology, enabling applications like atomic-scale nuclear magnetic resonance spectroscopy, and to read and write quantum information with nuclear spins in 2D materials.
|
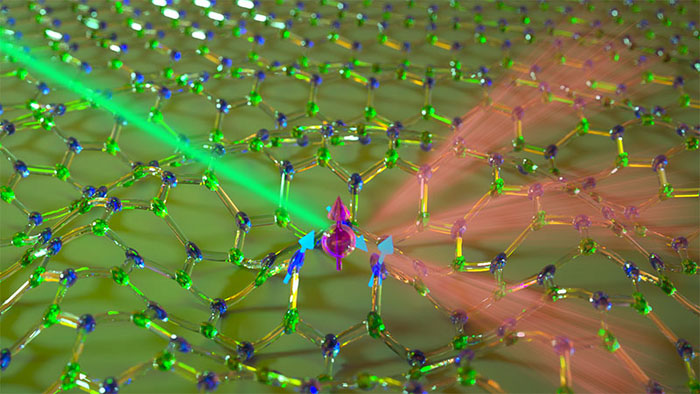 |
Researchers used light and electron spin qubits to control nuclear spin in a 2D material. (Image: Secondbay Studio)
|
Importance and Advantages of Spintronics
|
Spintronics is important because it offers several advantages over traditional electronics, including:
|
Energy efficiency: Spintronics technology has the potential to create non-volatile memory, meaning data would not be lost when the power is turned off. As a result, spintronics devices consume less power compared to traditional electronics, making them ideal for use in battery-operated devices. Spintronics devices consume less power compared to traditional electronics because they use the spin state of electrons to store information, instead of the charge state. The spin state of electrons is a quantum mechanical property and does not require any external power to maintain its state. This means that, once the spin state of the electrons has been set, it remains unchanged until it is changed by an external stimulus, like an electric or magnetic field, resulting in low power consumption compared to traditional electronics, where the charge state of the electrons needs to be actively maintained using power.
|
Higher storage density: Spintronics allows for the use of electron spin rather than charge to store data, which can result in a much higher data storage density. The reason scientists can pack more information into a smaller space by using the spin of electrons to store data is because they can have multiple stable spin states, each of which can represent a binary digit (e.g., 0 or 1). Traditional data storage methods use the charge of electrons, which has only two states, so there is a limit to how much information can be stored. However, with spintronics, multiple stable spin states allow for a much larger number of possible combinations, allowing for a much higher data storage density.
|
Enhanced speed and performance: Spintronics devices operate at faster speeds and have improved performance compared to traditional electronics – because the spin-based data transfer process is quicker and more efficient than charge-based data transfer – which can lead to faster and more efficient devices that makes them attractive for high-speed computing applications.
|
Improved durability: Because spintronics devices are less susceptible to data loss due to temperature fluctuations, magnetic fields, and other factors, they are less susceptible to damage from electromagnetic interference and heat, making them more durable. This has the potential to significantly increase the lifespan of electronic devices, which would lead to cost savings for consumers and businesses.
|
Versatility: In addition to applications in data storage, memory, and computing, the unique properties of spintronics also offer potential for new applications such as spintronic sensors, quantum cryptography, and quantum computing. This is because spintronics enables manipulation of quantum bits (qubits), which is essential for quantum information processing, and helps create quantum gates, which are critical components of quantum computers.
|
Examples of spintronic devices
|
Data storage and processing
|
Modern magnetic hard drives use read heads that take advantage of spin-related effects such as GMR and TMR. GMR devices utilize two or more layers of ferromagnetic materials separated by a spacer. When the magnetization vectors of the magnetic layers are aligned in the same direction, the electrical resistance is lower than when the vectors are in the opposite direction. This configuration is known as a spin valve. On the other hand, TMR devices achieve electron transport through the quantum mechanical tunneling of particles through an insulating layer separating the ferromagnetic layers.
|
GMR and TMR are phenomena that occur at the interface between different magnetic materials. In GMR, the electrical resistance of a structure changes depending on the relative orientation of the magnetization in the different magnetic layers. This change in resistance can be used to detect the magnetic field of a bit on a disk. In TMR, the electrical resistance of a structure changes depending on the relative orientation of the magnetization in the different magnetic layers and the electrons are able to tunnel through the insulating layer between the magnetic layers. This change in resistance can also be used to detect the magnetic field of a bit on a disk. These spin-related effects allow the read heads to detect the magnetic field of the bits on the disk with high sensitivity, and thus allows the hard drive to read the data stored on the disk with high accuracy.
|
There is a variety of spintronic devices that exploit the spin of electrons to store and process information:
|
Magnetic Random Access Memory (MRAM): This is a type of non-volatile memory that uses the magnetic moments of electrons to store data, rather than charge-based memory as in traditional RAM.
|
Spin Transfer Torque Random Access Memory (STT-RAM): This is a type of MRAM that uses the spin of electrons to switch the magnetic orientation of a magnetic material, resulting in a change in resistance that can be read as data.
|
Magnetic Tunnel Junctions (MTJs): This is a type of spintronic device that consists of two magnetic layers separated by an insulating layer. The spin-dependent transport of electrons through the insulating layer results in a change in resistance that can be used to store and read information.
|
Spin-Valve Sensors: These are sensors that use the spin-dependent transport of electrons to detect changes in magnetic fields. They are commonly used in hard disk drives to read the magnetic information stored on the disk.
|
Spin Hall Effect Devices: These are spintronic devices that use the spin-orbit interaction to generate a transverse spin current, which can be used to control the magnetic orientation of magnetic materials.
|
Magnetic Logic Devices: These are spintronic devices that use the magnetic orientation of magnetic materials to perform logical operations, such as AND, OR, and NOT. They have the potential to provide a new paradigm for computing that is faster, more energy-efficient, and more scalable than traditional charge-based computing.
|
Spin-Orbit Torque Devices: These are spintronic devices that use the spin-orbit interaction to generate a torque that can be used to switch the magnetic orientation of magnetic materials.
|
Spin-Valve Amplifiers: These are spintronic devices that use the spin-dependent transport of electrons to amplify electrical signals.
|
Magnetic Domain Wall Devices: These are spintronic devices that use the movement of magnetic domain walls in magnetic materials to store and process information.
|
Dzyaloshinskii-Moriya Interaction Devices: These are spintronic devices that use the Dzyaloshinskii-Moriya interaction, a type of spin-orbit interaction, to control the magnetic orientation of magnetic materials.
|
Topological Spintronics Devices: These are spintronic devices that exploit the unique properties of topological materials, such as topological insulators and Weyl semimetals, to generate, control, and manipulate spin currents.
|
Spin-Filter Devices: These are spintronic devices that use the spin-dependent transport of electrons to filter out electrons with a specific spin orientation.
|
Magnetic Sensors: These are spintronic devices that use the spin-dependent transport of electrons to detect changes in magnetic fields. They are commonly used in a wide range of applications, including navigation, medical imaging, and oil exploration.
|
Quantum computing
|
Spintronics can be used in quantum computing as a means of creating and manipulating qubits, which are the basic building blocks of a quantum computer. In quantum computing, qubits are used to represent and store information, and the manipulation of their quantum states is what allows quantum computers to perform certain calculations that are infeasible for classical computers.
|
Spintronics offers a promising approach to creating qubits because it allows for the manipulation of the spin of electrons, which can be used to represent and store quantum information. For example, a qubit can be implemented using a single electron spin in a magnetic material, where the spin state “up” represents a 0 and the spin state “down” represents a 1. The state of the electron spin can then be manipulated through magnetic fields or by applying voltage pulses.
|
Another example of using spintronics in quantum computing is the creation of a spin qubit using a ferromagnetic material sandwiched between two non-magnetic materials. The two non-magnetic materials act as spin filters that allow only one type of spin direction to pass through, while the ferromagnetic material acts as a spin rotator that can manipulate the direction of the spin. The resulting spin state of the electrons can then be read out by measuring the electrical resistance of the structure.
|
In both cases, spintronics provides a way to control and manipulate the spins of electrons, which can be used to create qubits and perform quantum computations. Although much work is still needed to make spintronics-based quantum computing a reality, the potential for spintronics to address some of the challenges faced by other qubit technologies makes it a promising area of research in the field of quantum computing.
|
Challenges in Developing Spintronics
|
While spintronics technology has the potential to revolutionize the field of electronics, there are several challenges that are currently holding back its commercial use. These include:
|
Materials challenges: Developing materials that can effectively manipulate the spin of electrons is a major challenge in the field of spintronics. The materials used in spintronics must have specific properties, such as high spin polarization and long spin relaxation times, in order to be effective. These properties are difficult to achieve and currently, there are only a few known materials that can be used for spintronics.
|
Manufacturing challenges: Manufacturing spintronics devices at a commercial scale is difficult because of the need to precisely control the properties of the materials used. The manufacturing process must be able to control the properties of the materials at a very small scale, which is difficult and costly.
|
Integration challenges: Integrating spintronics devices with traditional electronics is a major challenge. Spintronics devices use different operating principles than traditional electronics, which makes it difficult to integrate them into existing electronic systems.
|
Scalability challenges: Scaling up spintronics technology to commercial level is a challenge, as the devices are still at the experimental stage and need to be fine-tuned for industrial scale production.
|
Cost challenges: The materials and manufacturing processes used in spintronics are currently expensive, making it difficult to produce spintronics devices at a commercial scale.
|
Limited applications: While spintronics technology has a lot of potential, the current applications are limited, and it is not yet clear which specific areas of technology will be most impacted by spintronics.
|
Conclusion
|
The field of spintronics may seem complex and challenging to some due to its roots in quantum physics and chemistry. However, it is important to remember that electronics was once considered esoteric as well but is now a widely accepted and used technology that underpins modern society. Today, spintronics is a maturing field of applied science and engineering, as well as fascinating pure science in its own right. It is worth noting that spintronics is already established in one critical area of digital electronics: data storage, where the read heads of modern magnetic hard drives exploit the spin-related effects of Giant Magnetoresistance and Tunnel Magnetoresistance. As the field advances, it is becoming increasingly recognized for its potential to revolutionize information processing and storage.
|
By
Michael
Berger
–
Michael is author of three books by the Royal Society of Chemistry: Nano-Society: Pushing the Boundaries of Technology, Nanotechnology: The Future is Tiny, and Nanoengineering: The Skills and Tools Making Technology Invisible Copyright ©
Nanowerk
|
|