Inter-Individual Variations: A Challenge for the Standardisation of Complement Activation Assays
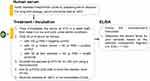
Dorelia Lipsa, Ana Ruiz Moreno, Cloé Desmet, Ivana Bianchi, Otmar Geiss, Pascal Colpo, Susanne Bremer-Hoffmann
Introduction: The role of the human immune system in pathologic responses to chemicals including nanomaterials was identified as a gap in current hazard assessments. However, the complexity of the human immune system as well as interspecies variations make the development of predictive toxicity tests challenging. In the present study, we have analysed to what extent fluctuations of the complement system of different individuals will have an impact on the standardisation of immunological tests.
Methods: We treated commercially available pooled sera (PS) from healthy males, individual sera from healthy donors and from patients suffering from cancer, immunodeficiency and allergies with small molecules and liposomes. Changes of iC3b protein levels measured in enzyme-linked immunosorbent assays served as biomarker for complement activation.
Results: The level of complement activation in PS differed significantly from responses of individual donors (p 0.01). Only seven out of 32 investigated sera from healthy donors responded similarly to the pooled serum. This variability was even more remarkable when investigating the effect of liposomes on the complement activation in sera from donors with pre-existing pathologies. Neither the 26 sera of donors with allergies nor sera of 16 donors with immunodeficiency responded similar to the PS of healthy donors. Allergy sufferers showed an increase in iC3b levels of 4.16-fold changes when compared to PS treated with liposomes.
Discussion: Our studies demonstrate that the use of pooled serum can lead to an over- or under-estimation of immunological response in particular for individuals with pre-existing pathologies. This is of high relevance when developing medical products based on nanomaterials and asks for a review of the current practice to use PS from healthy donors for the prediction of immunological effects of drugs in patients. A better understanding of individual toxicological responses to xenobiotics should be an essential part in safety assessments.
Graphical Abstract:
Keywords: inter-individual variability, complement activation, liposomes, standardisation, patients with pre-existing pathologies, healthy donors
Introduction
In the last decade, the paradigm shift in toxicology towards a better understanding of toxicological mechanisms for regulatory applications led to a number of major research projects including programs to develop tests based on human in vitro models.1 Unfortunately, a better understanding of individual susceptibilities that can affect toxic responses to environmental toxicants and drugs has been widely neglected. In particular, the role of the human immune system,2 its variability and contributions to pathologic responses to chemicals and pharmaceuticals have been flagged as a critical endpoint in hazard assessment. The upcoming revision of the classification, labelling and packaging of substances and mixtures (CLP) of EU-regulation will incorporate new hazard criteria that might also include the assessment of immunological effects.3 First indications on potential immune modulating effects can already be obtained from the standard toxicity studies (STS) in vivo that routinely investigate haematology, organ weights, and histological changes in lymphoid organs. However, such data are usually not sufficient to predict alterations of the human immune system and have to be complemented with studies following more specific guidelines/guidance (e.g., the harmonized tripartite guideline: immunotoxicity studies for human pharmaceuticals released by the International Council for Harmonization of Technical Requirements for Pharmaceuticals for Human Use (ICH)). Nevertheless, current regulatory practices do not adequately take into account interspecies variations and individual differences of the human immune system when they derive e.g. from genetic polymorphisms, age, environmental factors or the disease status. In vitro tests assessing the activation of the human complement systems are based on pooled sera from healthy donors and are well standardized.4 However, the pooling of sera from healthy donors bears the risk of underestimating the complement activation for certain genetic variants or vulnerable populations which can lead to the development of severe side effects.5,6 At the same time, an over-estimation of immune reactions can lead to the cancellation of a potentially valuable drug. It is therefore an open question to what extent the pooled serum from healthy donors will correctly predict the variability of individual immune responses. This can be critical for patients or vulnerable groups in the wider population that might react differently to nanotechnology-based health products e.g., drug delivery systems for anticancer drugs and vaccines. In order to overcome interspecies variations, new approach methodologies based on human in vitro models are currently being developed and standardised.7,8 Recent studies indicate that, measurements of C3 complement fragments may be a better indicator of human complement activation than cytotoxicity tests that are currently in use.9 In this study, we compared the complement activation by quantifying the iC3b complement fragments in pooled serum from healthy donors with the individual activation using sera from healthy donors and patients suffering from immune deficiencies, allergies and cancer. Nanomaterials in biomedical and pharmaceutical applications10–13 have become an innovation priority, particularly in diagnosis, implants, drug delivery systems, etc.14 In this study, we used commercially available empty liposomes as activators for the complement system in order to demonstrate the relevance of inter-individual variances for safety assessments of therapeutically relevant materials.15
Materials and Methods
Materials
Chemicals
In order to select the most reliable positive control, we used a series of different chemicals which are known to activate the complement system:16–18 Kolliphor EL (CAS 61791–12-6; code C5135-500G), Polyethylenimine, branched (bPEI, CAS 9002–98-6; code 408727–100mL), as well as to block the complement reaction Ethylenediaminetetraacetic acid disodium salt solution (EDTA, CAS 139–33-3; code 03690–100 mL) all chemicals purchased from Sigma Aldrich (Italy). The developed test system was exposed to therapeutically relevant liposomes purchased from FormuMax Scientific, Inc. (Palo Alto, CA, USA) having different surface functionalization, as indicated in the Supplements Table S1.
Biologicals
Pooled serum from healthy donors (cod A113, Quidel, Corporation, CA, USA) and individual serum samples derived from healthy donors as well as from patients suffering from diseases such as immunodeficiency, allergy and cancer (In.Vent, Germany) were aliquoted and stored at − 80°C until further analysis. Additional information on clinical parameters of human serum obtained from both healthy donors (nhealthy=32 donors) and patients with preconditions (nallergy=26, nimmunodeficinecy=16; ncancer=15) are presented in the Supplements Table S2. Heat Aggregated Gamma Globulin (HAGG; cat. A114) and Cobra Venom Factor (CVF; cat. A600) were purchased from Quidel (Santa Clara, CA, USA) while Zymosan A (CAS 58856–93-2) was purchased from Sigma Aldrich (Italy) to be used as positive controls for the activation of the complement system. The induction of iC3b levels were quantified with a commercially available Microvue Enzyme-linked Immunosorbent Assay kit (cat. A006, Quidel, Corporation, CA, USA) following manufacturer’s instructions.
Methods
The general experimental setup including physico-chemical characterization, potential contamination of selected nanoformulation as well as their biocompatibility with human serum is presented in Figure 1.
![]() |
Figure 1 Overview of the whole workflow in the experimental setup: Selection of complement activators; Quality assessment; Complement activation. |
![]() |
Figure 2 Serology testing protocol for evaluation of potential complement activation effects of test samples (ie, liposomes) in human serum. |
Physico-Chemical Characterization of Liposomes with Asymmetric Flow Field-Flow Fractionation Coupled with Dynamic Light Scattering (AF4-DLS)
The hydrodynamic diameter of the three selected liposomes (F20104, F20103, F10102) was measured by on-line coupling of asymmetric flow field flow fractionation (AF4) with dynamic light scattering (DLS). The AF4-system was composed of an Eclipse Dualtec separation system (Wyatt Technology Europe GmbH, Dernbach, Germany) and an Agilent 1260 Infinity high performance liquid chromatograph (Agilent Technologies, Santa Clara, USA) equipped with a degasser (G1322A), an isocratic pump (G1310B) and an autosampler (G1329B). In the Eclipse SC separation channel regenerated cellulose membranes (10 kDa) and a spacer height of 350 μm were used. The AF4 separation flow-parameters are included in the Supplementary Material Table S3. The AF4-system was coupled to a Malvern Zetasizer Nano-S DLS (Malvern Panalytical Ltd, United Kingdom), operated in flow-mode. Data were processed by the Zetasizer software version 7.11.
Working solutions of 500 ppm in PBS were prepared for each of the three liposomes. In a last step, the working solutions were diluted to reach 250 ppm before injection. One set of liposomes was diluted with PBS and one with diluted PBS (1:1 with ultrapure water). Before injection, these suspensions were incubated for 1h at 37 °C, following the experimental incubation conditions applied for the assessment of complement activation. The characterization of the selected liposomes in biologic relevant media has been carried out in our previous paper.19
Assay for Complement Activation Product iC3b
Before performing the complement activation assay, the levels of endotoxin contamination in the selected liposomes were quantified as presented in our recent work.19 Briefly, the endotoxin levels were determined using the Limulus amoebocyte lysate (LAL) chromogenic methods by kinetic assay. The control standard curve was prepared with the standard endotoxin from the E. coli O111:B4 strain (cat. EC010, 10 ng/vial; Associates of Cape Code, Inc.). The sample readouts were carried out at 405 nm, according to manufacturer’s instruction. Results for each sample were taken into consideration only if the correlation coefficient of the calibration curve was ≥0.98.
A previously published protocol for the quantitative determination of complement activation in human plasma was further optimised as described in Figure 2.20
The liposome’s stock solutions were stored at 4 °C. Working solutions of 450 μg/mL and 150 μg/mL were prepared by diluting the liposomes in sterile PBS buffer (pH 7.4). The liposome solutions for in vitro testing were prepared at concentrations of 50 and 150 μg/mL by mixing the liposomes working solutions with PBS and human serum. In brief, the enzyme-linked immunosorbent assay (ELISA) was performed according to the MicroVue iC3b EIA Quidel manufacturer’s instructions. Serum samples were diluted in a U-shaped 96-well plate by 1:100 in the specimen diluent. Then the standards, the controls and the diluted serum samples were transferred to the ELISA plate and incubated at room temperature for 30 min. The ELISA plate was thoroughly washed with wash solution provided by the kit. The iC3b conjugate was added to the plate for 30 min and after washing steps, substrate solution was added to the plate for 30 min. Finally, the addition of stop solution blocked the enzymatic reaction and the iC3b levels were determined by absorbance reading at 405 nm with an EnSpire® Multimode plate reader (Perkin Elmer).
Statistical Analysis
Each experiment was performed in two technical replicates and three independent runs. The level of complement activation for each experiment is expressed as M ± σM, where M is the average of the mean values obtained in each independent run and σM the average standard deviation calculated by:
To calculate the activation of the complement system, the concentration of iC3b measured in the negative control (PBS-challenged sera) was subtracted from the concentration measured in the tested samples (cobra venom and liposomes-challenged sera). The standard variation of the subtraction was calculated as the square root of the sum of the quadrature of the standard deviation of the negative control plus the quadrature of the standard deviation of the test samples. Statistical comparison among the different groups was carried out by running a one-way analysis of variance (ANOVA) and Dunnett’s Multiple comparison test. Differences were considered as statistically significant among the selected groups when p < 0.05.
Results
Liposomes Size and Stability Characterization by AF4-DLS
The stability and size of the investigated liposomes were characterized by using AF4-DLS. The z-average diameter of all three particles ranged from approximately 75 to approximately 120 nm, confirming the sizes specified by the purchaser (Table S2). This indicates that no relevant size-shift occurred during incubation with PBS, diluted PBS and assay medium (see Supplementary Figure S1).
Optimization of Complement Assay Performance
Currently, various methods using iC3b as biomarker for the activation of the complement system exist. We have adapted an existing protocol by defining incubation times (30 and 60 minutes) for optimal iC3b generation levels and compared the potency of five different positive control substances to activate the iC3b complement product. In addition, we have analysed three different buffers: Ca2+/Mg2+ free Phosphate-buffered saline (PBS), Hank’s balanced salt solution (HBSS) and Limulus Amebocyte Lysate reagent water (LAL H2O) which were used for the dilution of the serum as potential negative controls (Figure 3).
![]() |
Figure 3 iC3b levels measured in pooled human serum incubated with positive and negative controls. |
The synthetic molecules bPEI (0.2 mg/mL) and Kolliphor (0.2%) showed higher levels of the complement fragment iC3b compared to the selected natural stimulants eg Zymosan A (1.3 mg/mL), Heat Aggregated Gamma Globulin (HAGG) (1.3 mg/mL) and CV using (10 units/mL). Even if Branched Polyethylenimine (bPEI) was the most potent substance, we selected Cobra Venom (CV) as the positive control because of the relatively low variability between independent experiments.
iC3b Variability in Sera from Healthy Donors
In order to better understand the relevance of individual responses for the standardizstion of an in vitro test, we have treated 32 sera of healthy donors with 10 units/mL of CV and compared the individual responses with human pooled serum currently used in established protocol (Figure 4). Whereas pooled serum induced an average of 193 ± 15 µg/mL of iC3b (horizontal grey bar), individual responses ranged from 105 µg/mL – 288 µg/mL. Only seven out of 32 donors responded similarly to the pooled serum. Nine donors had lower induction values and 16 donors had higher induction values. The trend of higher iC3b values in sera of donors with blood type B is interesting but would require an enlargement of the sample group to proof statistical significance (Figure 4). However, the mean value of the individual responses (virtual pooling) is in the margin of pooled serum (see pink cross in Figure 4). The mean values of the different blood types also fall within the pooled serum average except for blood type B, which presents higher value. We could not observe differences in responses with regard to gender and RH variability (Figure S2 Supplements).
iC3b Variability in Sera from Donors with Pre-Existing Conditions
In order to understand the relevance of the inter-individual variability of donors with pre-existing conditions, we have analysed the amount of iC3b in sera of patients suffering from immune deficiencies, allergies or cancer. The response in 26 patients with allergies was very variable ranging from 225 µg/mL to 911 µg/mL. The iC3b induction in sera deriving from 16 immunodeficient patients was lower than iC3b levels measured in human pooled serum varying from 43 to 59 µg/mL. The iC3b levels measured in serum from 15 patients suffering from cancer are slightly lower than pooled serum.
Interestingly, none of the investigated sera responded similarly to the sera of pooled serum of healthy donors (193 ± 15 μg/mL) (Figure 5A). A trend towards higher iC3b induction values in sera from males with allergies (Figure 5B) was observed. Other parameter such as blood-type and RH factor did not show any significant differences (data not shown). A correlation between the activation of the complement system and the type of allergy type could not be established with the obtained data.
Variations in iC3b Levels Triggered by Liposomes in Healthy Donors and Donors with Preconditions
In a following step, we analysed the relevance of inter-individual variations for the prediction of potential safety issues of materials with therapeutic relevance by assessing the activation of the complement system triggered by liposomes with different physico-chemical characterizations (Table S1).
The dose response curves show that the liposome F20104A activated the complement system in a concentration-depending manner in pooled serum (Figure 6A). This liposome was selected as a benchmark material. Measurement of iC3b induction values by using therapeutically relevant concentrations 150 μg/mL20 and 50 μg/mL of the liposome F20104A demonstrated substantial variations of individual responses, which do not correspond to the iC3b levels quantified in pooled serum (Figure 6B). For the liposome tested in pooled serum at 50 μg/mL, the average is 29.77 ± 27.2µg/mL, while for the liposome tested at 150 ug/mL the average is 146.27 ± 9.35 µg/mL.
The differences in gender and RH did not affect the variations of iC3b responses (Figure S3 Supplements).
In successive studies, we studied the individual activation of the complement system in sera from donors with and without pre-existing conditions by quantifying the amount of iC3b. In particular, the physiologically relevant concentration of 150 µg/mL F20104A showed a significant variability of individual responses when compared to pooled serum of healthy donors (p < 0.01 for blood types A, B and AB; p < 0.05 for blood type O). Only 1 out of 32 donors would have similar iC3b values as those measured in pooled serum. At a lower concentration (50 µg/mL) the number of donors is increasing to 16 (Figure 7). Patients suffering from allergies showed a 4.16 fold increase in iC3b levels when compared to pooled serum treated with liposomes (p < 0.001 for all blood types) whereas immunocompromised patients showed a decrease in the iC3b fragment complement (Figure 7). The average of the iC3b activation of serum from 15 cancer patients (141 ± 8 µg/mL) was within the average of the response of pooled serum of healthy donors (146 ± 9 µg/mL).
Discussion
Safety assessments of nanotechnology-based health products can be challenging because they frequently trigger immunological responses.21 Existing animal tests are often not very predictive and are associated with uncertainties due to interspecies variations.13 In order to address these shortcomings in regulatory science, a number of in vitro methods based on human models are currently being developed and standardized22 but without considering individual differences of immune responses. With the aim to better understand the biological relevance of inter-individual variances for safety assessments of nanomedicines, we compared the activation of the complement system of individual healthy donors with donors having pre-existing pathologies. The mechanism of complement activation is well understood and23 various complement fragments including iC3b24–26 can be easily detected in human plasma and/or serum.27–30 A range of assays to analyse the human complement system have been developed.31 Most common examples are immunoprecipitation assays or enzyme immunoassays (EIA). The measurement of iC3b complement fragments by EIA is a rapid and non-radioactive method, a relevant tool that is widely applied in both research and clinical studies. Commercially available test kits have been effectively applied in biomedical research and more specifically in non-clinical safety assessments of nanotechnology-based health products. In the current standardized test protocol, a 2-fold increase of iC3b complement fragments compared to the negative control is considered as an activation of the complement system.32–34 However, these tests are using pooled sera from healthy donors in order to obtain reliable results within and between laboratories.35 Nevertheless, our analysis showed individual differences between the donors and in particular sera from patients with allergies showed an increased activation of the complement system compared to pooled sera. In consequence, immune responses of individuals with allergies would be under-estimated when pooled sera from healthy donors is used; whereas immunological reactions of immune compromised individuals would be over-estimated.
Benasutti et al already reported on variations in the activation of the complement systems of healthy donors without statistical difference between males and females,- an observation which is confirmed with our analysis.36 Temporal response changes are another highly important parameter adding to the complexity for standardization of a relevant assay.37,38 However, variations in donors with pre-existing conditions when treated with therapeutically relevant drug delivery systems have not been investigated to our knowledge. Our results suggest that sera used in predictive tests should reflect the inter-individual variations and should be selected depending on the future use of the investigated formulation e.g., a drug delivery system or vaccines that will be applied to a wider population with very different immunological profiles. Moreover, the presented data show that a biological test system based only on sera from healthy donors would eventually under-estimate potential immune responses whereas liposomal product administered to immune suppressed patients will over-estimate reactions. The over-estimation of side effects in the preclinical phase might lead to the termination of valuable drugs whereas the under-estimation of hazards of an in vitro test would put individuals at risk. Our study suggests considering the development of prior diagnostic tools accompanying innovative health products for vulnerable populations in particular when new formulations are known to interact with the human immune system.
In addition, these preliminary results demonstrate the capability of in vitro tests based on human materials to support the understanding of inter-individual variations, which cannot be obtained from animal experiments. This is of particular importance for toxicological areas where a high inter-individual variability is expected. Even if more studies are necessary, our results already support a need for an in-depth discussion across industrial sectors on the relevance of inter-individual immune responses for the standardisation of predictive in vitro assays. Progresses in regulatory science should include a review on the current practice to address inter-individual variations with a factor of 10 as a margin of safety in particular when it comes to toxicological areas with significant inter-individual variations such as immunotoxicity. In addition, the identification of vulnerable populations grouped on their immune status should be of high importance also in the non-pharmacological sectors.
Acknowledgments
The work was performed in the frame of the JRC Exploratory Research project “Personalised Immunological Risk Assessment Technologies” (PIRAT) and partially supported by the REFINE project (grant agreement no Horizon 2020, Grant Agreement no 761104). We thank Douglas Gilliland and Barbara Erskine for proofreading our paper.
Disclosure
The authors report no conflicts of interest in this work. The abstract of this paper was presented at the One EU Conference 2022 as an E-poster with interim findings. The poster’s abstract was published online in “Poster Abstracts” under the thematic tracks “Many ways” and thematic session “New approach methodologies: moving beyond animal testing” and can be accessed at https://www.one2022.eu/posters/abstracts.
The human sera obtained from In.vent Diagnostica/Germany and Quidel/US comply with European ethical standards as requested by the Declaration of Helsinki (https://inventdiagnostica.de/en/company/). The whole study was reviewed by JRC permanent Research Ethics Board (evaluation id: 30888_1_18112022).
References
1. Sun H, Xia M, Austin CP, Huang R. Paradigm shift in toxicity testing and modeling. AAPS J. 2012;14(3):473–480. doi:10.1208/s12248-012-9358-1
2. Liston A, Humblet-Baron S, Duffy D, Goris A. Human immune diversity: from evolution to modernity. Nat Immunol. 2021;22(12):1479–1489. doi:10.1038/s41590-021-01058-1
3. Revision of EU legislation on hazard classification, labelling and packaging of chemicals. Available from: https://ec.europa.eu/info/law/better-regulation/have-your-say/initiatives/12975-Revision-of-EU-legislation-on-hazard-classification-labelling-and-packaging-of-chemicals_en. Accessed May 20, 2022.
4. Hempel JC, Poppelaars F, Gaya da Costa M. Distinct in vitro complement activation by various intravenous iron preparations. Am J Nephrol. 2017;45(1):49–59. doi:10.1159/000451060
5. de Jong S, Gagliardi G, Garanto A, et al. Implications of genetic variation in the complement system in age-related macular degeneration. Prog Retin Eye Res. 2021;84:100952. doi:10.1016/j.preteyeres.2021.100952
6. Zhang J, Li S, Hu S, Yu J, Xiang Y. Association between genetic variation of complement C3 and the susceptibility to advanced age-related macular degeneration: a meta-analysis. BMC Ophthalmol. 2018;18(1):274. doi:10.1186/s12886-018-0945-5
7. Parish ST, Aschner M, Casey W, et al. An evaluation framework for new approach methodologies (NAMs) for human health safety assessment. Regul Toxicol Pharmacol. 2020;112:104592. doi:10.1016/j.yrtph.2020.104592
8. Niethammer M, Burgdorf T, Wistorf E, Schönfelder G, Kleinsorge M. In vitro models of human development and their potential application in developmental toxicity testing. Development. 2022;149(20):dev200933. doi:10.1242/dev.200933
9. Watanabe J, Scornik JC. Measuring human complement activation by HLA antibodies. Arch Pathol Lab Med. 2006;130(3):368–373. doi:10.5858/2006-130-368-MHCABH
10. Mabrouk M, Das DB, Salem ZA, Beherei HH. Nanomaterials for biomedical applications: production, characterisations, recent trends and difficulties. Molecules. 2021;26(4):1077. doi:10.3390/molecules26041077
11. Ramos AP, Cruz MAE, Tovani CB, Ciancaglini P. Biomedical applications of nanotechnology. Biophys Rev. 2017;9(2):79–89. doi:10.1007/s12551-016-0246-2
12. Khatua A, Priyadarshini E, Rajamani P, et al. Phytosynthesis, characterization and fungicidal potential of emerging gold nanoparticles using pongamia pinnata leave extract: a novel approach in nanoparticle synthesis. J Clust Sci. 2020;31(1):125–131. doi:10.1007/s10876-019-01624-6
13. Barabadi H, Tajani B, Moradi M, et al. Penicillium family as emerging nanofactory for biosynthesis of green nanomaterials: a journey into the world of microorganisms. J Clust Sci. 2019;30(4):843–856. doi:10.1007/s10876-019-01554-3
14. Aygün A, Gülbağça F, Nas MS, et al. Biological synthesis of silver nanoparticles using Rheum ribes and evaluation of their anticarcinogenic and antimicrobial potential: a novel approach in phytonanotechnology. J Pharm Biomed Anal. 2020;179:113012. doi:10.1016/j.jpba.2019.113012
15. Halamoda‐Kenzaoui B, Holzwarth U, Roebben G, Bogni A, Bremer‐Hoffmann S. Mapping of the available standards against the regulatory needs for nanomedicines. WIREs Nanomed Nanobiotechnol. 2019;11(1). doi:10.1002/wnan.1531
16. Szebeni J. Complement activation-related pseudoallergy: a stress reaction in blood triggered by nanomedicines and biologicals. Mol Immunol. 2014;61(2):163–173. doi:10.1016/j.molimm.2014.06.038
17. Merkel OM, Urbanics R, Bedőcs P, et al. In vitro and in vivo complement activation and related anaphylactic effects associated with polyethylenimine and polyethylenimine-graft-poly(ethylene glycol) block copolymers. Biomaterials. 2011;32(21):4936–4942. doi:10.1016/j.biomaterials.2011.03.035
18. Jackman JA, Mészáros T, Fülöp T, Urbanics R, Szebeni J, Cho NJ. Comparison of complement activation-related pseudoallergy in miniature and domestic pigs: foundation of a validatable immune toxicity model. Nanomedicine. 2016;12(4):933–943. doi:10.1016/j.nano.2015.12.377
19. Della Camera G, Lipsa D, Mehn D, et al. Approach to improve clinical translation of liposome-based nanomaterials, a focus on innate immune and inflammatory responses. IJMS. 2021;22(2):820. doi:10.3390/ijms22020820
20. Neun B, Cedrone E, Dobrovolskaia M. NCL Method ITA-5.2. Available from: https://ncihub.org/publications/204/supportingdocs?v=2. Accessed May 20, 2022.
21. Halamoda-Kenzaoui B, Bremer-Hoffmann S. Main trends of immune effects triggered by nanomedicines in preclinical studies. IJN. 2018;13:5419–5431. doi:10.2147/IJN.S168808
22. Hartung T, Daston G. Are in vitro tests suitable for regulatory use? Toxicol Sci. 2009;111(2):233–237. doi:10.1093/toxsci/kfp149
23. Wagar LE, DiFazio RM, Davis MM. Advanced model systems and tools for basic and translational human immunology. Genome Med. 2018;10(1):73. doi:10.1186/s13073-018-0584-8
24. Kim AHJ, Strand V, Sen DP, et al. Association of blood concentrations of complement split product iC 3b and serum C3 with systemic lupus erythematosus disease activity. Arthritis Rheumatol. 2019;71(3):420–430. doi:10.1002/art.40747
25. Capecchi R, Puxeddu I, Pratesi F, Migliorini P. New biomarkers in SLE: from bench to bedside. Rheumatology. 2020;59(Supplement_5):v12–v18. doi:10.1093/rheumatology/keaa484
26. Schramm EC, Staten NR, Zhang Z, et al. A quantitative lateral flow assay to detect complement activation in blood. Anal Biochem. 2015;477:78–85. doi:10.1016/j.ab.2015.01.024
27. Sandholm K. Development and Evaluation of Immunoassays for Complement Diagnostics [Thesis]. Linnaeus University, Faculty of Health and Life Sciences, Department of Chemistry and Biomedical Sciences; 2019. Available from: http://urn.kb.se/resolve?urn=urnnbn:se:lnu:diva-90125:. Accessed May 20, 2022.
28. Peerschke EI, Valentino A, So RJ, Shulman S. Thromboinflammation supports complement activation in cancer patients with COVID-19. Front Immunol. 2021;12:716361. doi:10.3389/fimmu.2021.716361
29. Sharif SN, Goldberg B. Investigation of complement activation in patients with chronic idiopathic urticaria. J Allergy and Clin Immunol. 2007;119(1):S200. doi:10.1016/j.jaci.2006.12.151
30. Java A, Baciu P, Widjajahakim R, et al. Functional analysis of rare genetic variants in complement factor I (CFI) using a serum-based assay in advanced age-related macular degeneration. Trans Vis Sci Tech. 2020;9(9):37. doi:10.1167/tvst.9.9.37
31. Skattum L. Clinical complement analysis—an overview. Transfus Med Rev. 2019;33(4):207–216. doi:10.1016/j.tmrv.2019.09.001
32. Caputo F, Clogston J, Calzolai L, Rösslein M, Prina-Mello A. Measuring particle size distribution of nanoparticle enabled medicinal products, the joint view of EUNCL and NCI-NCL. A step by step approach combining orthogonal measurements with increasing complexity. J Control Release. 2019;299:31–43. doi:10.1016/j.jconrel.2019.02.030
33. Liptrott N. Analysis of complement activation by EIA. Available from: https://www.euncl.org/about-us/assay-cascade/PDFs/Haematology/EUNCL-ITA-005_2.pdf?m=1526624464&. Accessed May 20, 2022.
34. Neun B, Ilinskaya A, Dobrovolskaia M. NCL Method ITA-5.1. Available from: https://ncihub.org/publications/133/supportingdocs?v=3. Accessed May 20, 2022.
35. Bergseth G, Ludviksen JK, Kirschfink M, Giclas PC, Nilsson B, Mollnes TE. An international serum standard for application in assays to detect human complement activation products. Mol Immunol. 2013;56(3):232–239. doi:10.1016/j.molimm.2013.05.221
36. Benasutti H, Wang G, Vu VP, et al. Variability of complement response toward preclinical and clinical nanocarriers in the general population. Bioconjugate Chem. 2017;28(11):2747–2755. doi:10.1021/acs.bioconjchem.7b00496
37. Brodin P, Davis MM. Human immune system variation. Nat Rev Immunol. 2017;17(1):21–29. doi:10.1038/nri.2016.125
38. Lakshmikanth T, Muhammad SA, Olin A, et al. Human immune system variation during 1 year. Cell Rep. 2020;32(3):107923. doi:10.1016/j.celrep.2020.107923