Batteries not included: How your own body could power wearables
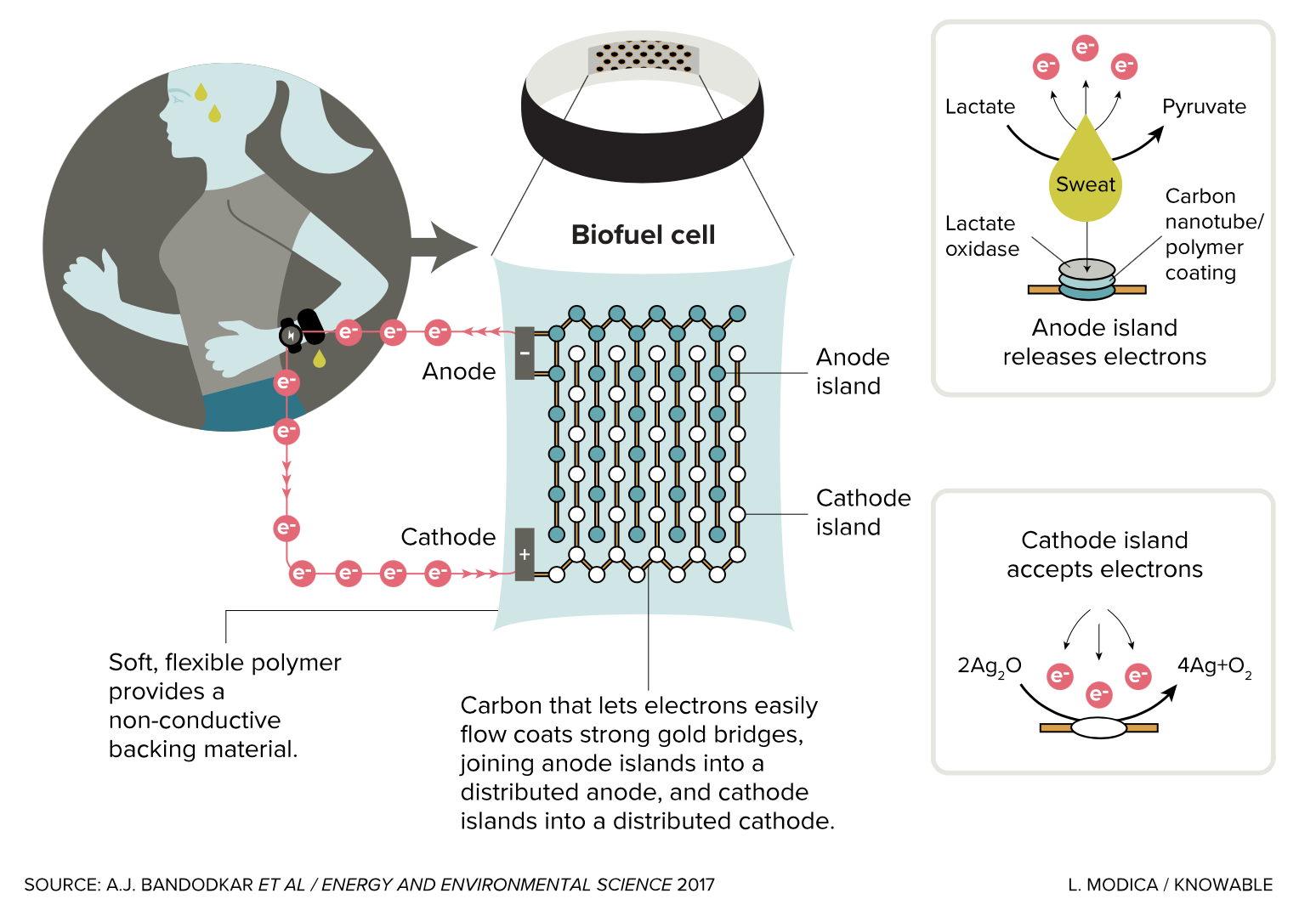
<?xml encoding=”utf-8″ ?????>
In “I Sing the Body Electric,” poet Walt Whitman waxed lyrically about the “action and power” of “beautiful, curious, breathing, laughing flesh.” More than 150 years later, MIT materials scientist and engineer Canan Dagdeviren and her colleagues are giving new meaning to Whitman’s poem with a device that can generate electricity from the way it distorts in response to the beating of the heart.
Electronics are now so powerful that a smartphone has more computing power than all of NASA did when it put the first men on the moon in 1969. The extraordinary advances technology has made over time have raised hopes that devices worn on, or even implanted within, the body can also become ever more capable.
A key drawback of most wearable and implantable devices still remains their batteries, whose limited capacities restrict their long-term use. The last thing you want to do when, say, a pacemaker runs out of power is to open up a patient just for a battery replacement. The solution to this problem may rest inside the human body — rich as it is in energy, in its chemical, thermal and mechanical forms. This has led scientists to investigate a plethora of ways for devices to harvest energy from the bodies that host them, detailed by Dagdeviren and her colleagues in the 2017 Annual Review of Biomedical Engineering.
The bellows-like motions that a person makes while breathing, for example, can generate 0.83 watts of power; the heat from a person’s body, up to 4.8 watts; and the motions of a person’s arms, up to 60 watts. That’s not nothing when you consider that a pacemaker needs just 50 millionths of a watt of power, a hearing aid needs a thousandth of a watt, a smartphone requires merely one watt.
Now Dagdeviren and others are designing machines that use the human body itself as their source of energy. Increasingly, researchers are testing such wearable or implantable devices in animal models and people.
Good vibrations
One energy-harvesting strategy involves converting energy from vibrations, pressure and other mechanical stresses to electrical energy. This approach, producing what is known as piezoelectricity, is often used in loudspeakers and microphones.
A commonly used piezoelectric material is lead zirconate titanate, whose lead content raises concerns that it might prove too toxic for use with humans. “But for lead to decompose from the structures, they would have to be heated to temperatures higher than 700°C,” Dagdeviren says. “You’ll never reach such temperatures in the body.”
To take advantage of piezoelectricity, Dagdeviren and her colleagues have developed flat devices that can be stuck onto organs and muscles such as the heart, lungs and diaphragm. These devices are “mechanically invisible” in that their mechanical properties are similar to whatever they are laminated onto, so they don’t hinder those tissues when they move.
So far, such devices have been tested in cows, sheep and pigs, all animals with hearts roughly the same size as those of people. “When these devices mechanically distort, they create positive and negative charges, voltage and current — and you can collect this energy to recharge batteries,” Dagdeviren explains. “You can use them to run biomedical devices like cardiac pacemakers instead of changing them every six or seven years when their batteries are depleted.”
Scientists are also developing wearable piezoelectric energy harvesters that can be worn on joints such as the knee or elbow, or in shoes, trousers or underwear. That way, a person can generate electricity for electronics whenever they walk or bend their arms.
When designing piezoelectric gadgets, one might — counterintuitively — not want the materials that are best at generating electricity. Instead of choosing a material that converts 5 percent of all mechanical energy it receives into electricity, for example, you might want a material with only 2 percent or less efficiency. If it converts more, “it might do so by placing more of a load on the body, and you don’t want it to make you tired,” Dagdeviren says.
Body heat
A different energy-harvesting approach uses thermoelectric materials to convert body heat to electricity. “Your heart beats more than 40 million times a year,” Dagdeviren notes. All that energy is dissipated as heat in the body — it’s a rich potential source to capture for other uses.
Thermoelectric generators do face some key challenges. They rely on temperature differences, but people usually keep a fairly constant temperature throughout their bodies, so any temperature differences found within are generally not dramatic enough to generate large amounts of electricity. But this is not a problem if the devices are exposed to relatively cool air in addition to the body’s continuous warmth.
Scientists are exploring thermoelectric devices for wearable purposes, such as powering wristwatches. In principle, the heat from a human body can generate enough electricity to power wireless health monitors, cochlear implants and deep-brain stimulators to treat disorders such as Parkinson’s disease.
Static and dynamic
Scientists have also sought to use the same effect behind everyday static electricity to power devices. When two different materials repeatedly collide with, or rub against, one another, the surface of one material can steal electrons from the surface of the other, accumulating a charge, a phenomenon known as triboelectricity. A key virtue of triboelectricity is that nearly all materials, both natural and synthetic, are capable of creating it, giving researchers a wide range of possibilities from which they can design gadgets.
“The more I work with triboelectricity, the more exciting it gets, and the more applications it might have,” says nanotechnologist Zhong Lin Wang at Georgia Tech, the review’s co-author. “I can see myself devoting the next 20 years to it.”
The surfaces selected for use in triboelectric devices can make a great difference in how much electricity they produce, so scientists are experimenting with a variety of forms and textures. Researchers have fabricated grids of cubes resembling microscopic city blocks, fields of nanowires resembling bamboo forests, and arrays of pyramids resembling those found in Giza. These materials not only “look beautiful,” Wang says, but covering a surface with pyramids can boost the amount of electricity generated by a factor of five compared to just having a flat sheet.
Pacemakers, heart monitors and other implantable devices triboelectrically powered by breathing and heartbeats have been tested in rats, rabbits and pigs. “We’re also seeing if triboelectricity can be used inside the body to stimulate cell growth and enhance healing of wounds,” Wang says. “In addition, we’ve started experiments with triboelectricity on nerve stimulation, to see if we can contribute anything to neuroscience.”
Wang and his colleagues have also designed triboelectrically powered wearable devices. For instance, they fabricated triboelectric cloth that can recharge a flexible belt containing lithium-ion batteries. That can power a wearable heartbeat meter that uses Bluetooth technology to wirelessly send its data to a smartphone. “The mechanical energy of daily human motions can get converted into electricity by our cloth,” Wang says.
Blood and sweat
Another strategy relies on devices known as biofuel cells that generate electricity via chemical reactions between enzymes and fuel molecules that are in the body, such as glucose in the blood, or are released from the body, such as lactate secreted in sweat. For example, the enzyme cellobiose dehydrogenase from the fungus Phanerochaete sordida can break down sugars and generate electrical current when stuck onto carbon tubes only nanometers (billionths of a meter) wide.
The choice of enzyme can prove tricky. For instance, while several groups of scientists have found that glucose oxidase can produce electricity in biofuel cells implanted in lab rats, the enzyme also generates hydrogen peroxide, a common bleach. That can deteriorate the performance of the device and prove harmful to the body in the long term.
Scientists in France have created a biofuel cell based on enzyme-coated carbon nanotubes that is roughly only half a teaspoon in volume, and when implanted in rats, it can generate enough electricity from blood sugar to power an LED or a digital thermometer. Experiments have also shown that biofuel cells woven into garments such as headbands and wristbands can readily generate enough electricity from chemical reactions with sweat lactate to power a watch.
<?xml encoding=”utf-8″ ?????>
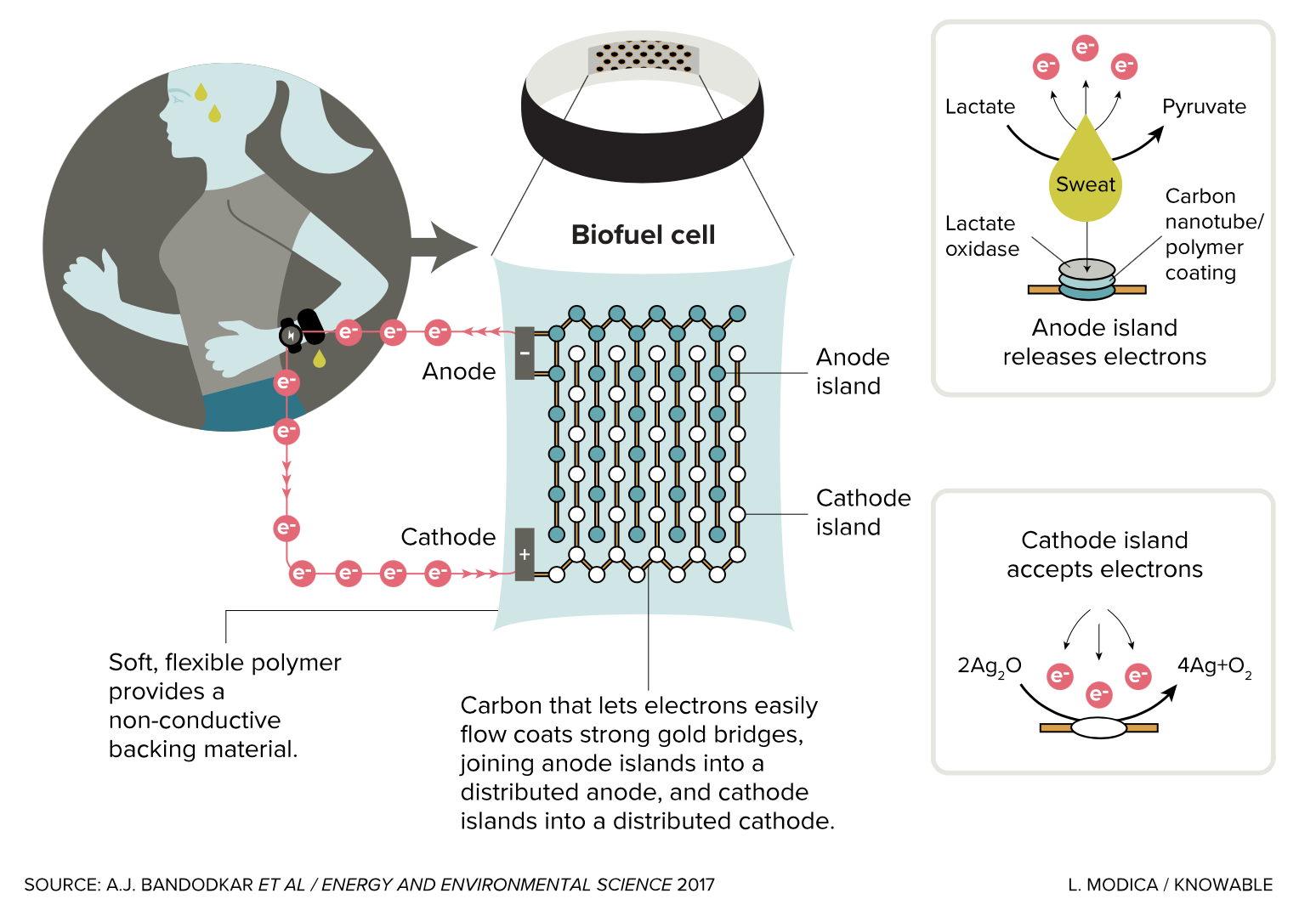
None of these devices are on the market yet, as far as Dagdeviren knows. But it might be less than 10 years before they start appearing for sale, she predicts. And in the future, energy-harvesting devices may become better tailored for the body. Dagdeviren and her colleagues are even investigating dissolvable versions of their gadgets.
“Imagine,” she says, “having a device inserted into your body, and after it works for a while, it dissolves away down to the molecular level in your bodily fluids. And you don’t have to open your chest to take it out: We can use biodegradable materials such as silk and zinc oxide that can break down over time.”
This article originally appeared in Knowable Magazine, a nonprofit publication dedicated to making scientific knowledge accessible to all. Sign up for Knowable Magazine’s newsletter.