An interview with AES Mid-Career Award recipient Jason Dwyer
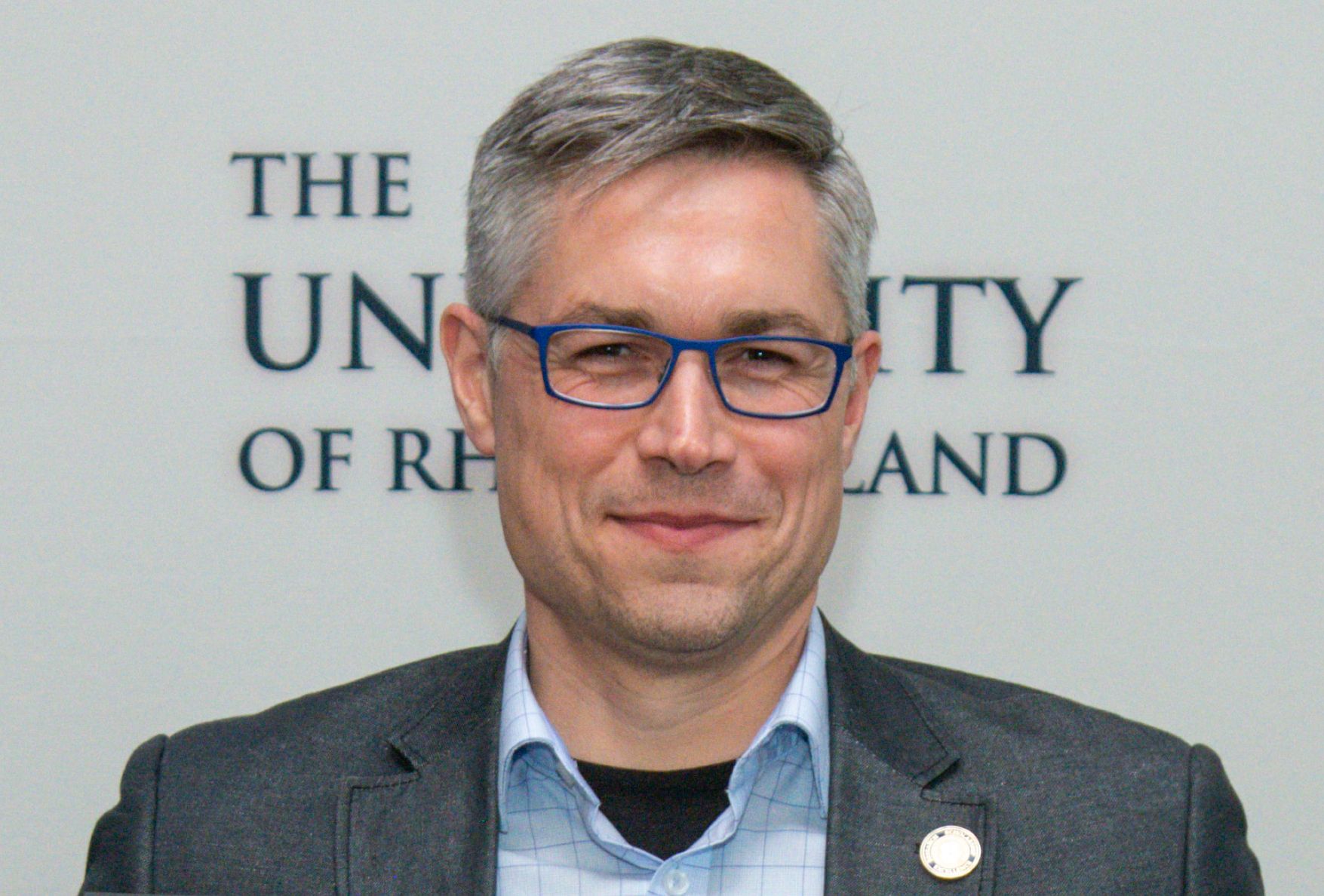
Jason Dwyer of the University of Rhode Island has been named the recipient of the American Electrophoresis Society’s Mid-Career Award, which honors exceptional contributions to the field of electrophoresis, microfluidics, and related areas by an individual who is currently in the middle of their career. Dwyer will be receiving the award at this year’s SciX conference, to be held from October 20 through October 25, in Raleigh, North Carolina. As part of an ongoing series of this year’s SciX conference honorees, Jason took some time to speak to Spectroscopy Magazine about his career so far, and where he sees the road forward leading him.
On your university webpage (1), you and your team are credited as “developing tools that can detect one molecule at a time.” Was science in general (and the concept of molecules specifically) something that you were interested in at an early age?
I’ve been drawn to science since Grade 1 and fascinated by molecules since I learned about them. I love the tools of physics and the complexity and importance of biology. Nanotechnology still amazes me. And yet it’s always been the molecular scale and perspective of chemistry that has most captivated my attention. The ability to control and exploit form, function, and properties on the sub-Angstrom scale is awe-inspiring. Of course, that’s not to say that I didn’t spend high school contemplating a path to hosting duties on “Jeopardy,” or that the humanities don’t continue to beckon me. Thankfully, the lectern and library provide ample ongoing opportunities to explore all these interests.
Your research group website (2) states that you are interested in “materials-science approaches for improving surface-enhanced Raman spectroscopy (SERS) flexibility and performance while augmenting the functions of the support material to have SERS capabilities.” What makes SERS a favored technique for your work?
Our interest in SERS is a happy accident of our materials science work and an unexpected return to a spectroscopy method I’d worked on as an undergraduate researcher in the laboratory of Professor Kathleen Gough (University of Manitoba). I’d actually been out of optics for quite a few years doing work in non-optical sensor development. In doing that work, we created nanostructured surfaces that were well-suited to a host of SERS-related applications and investigations. This happy return to optics has repeated itself across several different materials science projects. What I like about SERS is the flexibility of optics, the ability to fingerprint molecules even at low concentrations, and the close connection between materials chemistry, nanoscale fabrication, and application. I also kind of like that a lot of things can go wrong if you’re not careful. That means that you then get to accept the challenge and play the game—at the molecular or device level—of making sure those problems don’t happen.
I had taken up my assistant professorship with a focus on non-optical biomedical sensing using nanopores. Unfortunately, the university didn’t have the instrumentation needed to make them—probably the biggest risk I’ve taken in my career! So, instead, we bought micropores and then shrunk them down to the nanoscale using electroless gold plating (3). (Interestingly, I’ve met several industry researchers surprised to see this technique used in academia. Electroless plating is also used in art world, and it delights me when art—home to some of the first materials scientists—provides inspiration, or even just the answer, for science). SERS was also the fruitful outlet of a far more playful project. We had bought a $20 flameless cigarette lighter, essentially a handheld battery-powered Tesla coil (4,5), and then just started to see what it would do to the different microfabrication materials we work with. It turns out, for reasons we haven’t systematically studied, that it left us with micro- and nanostructured silicon that we could then coat with gold to use as a low-cost monolithic SERS substrate.
Briefly discuss some of your efforts in the improvement of the SERS technique.
We like to make targeted contributions where we think our perspective or capabilities can have an impact and serve others. While we have done some fundamental work, we generally approach this area with most of our attention focused on end-use considerations such as lower cost, streamlined operation, ease-of-use, robustness, reproducibility, and tool development. I thought that a lot of the most accessible background subtraction methods were problematic and limiting on several fronts, and so explored the performance, for several spectroscopies, of an open-source wavelet-based method that had been developed for high-throughput background subtraction in time-resolved diffraction (6). We also tackled background “subtraction” in the laboratory by exploring oxygen plasma cleaning of high-performance nanopillar SERS substrates. Without care, oxygen plasma cleaning can significantly degrade the SERS performance via unexpected structural modifications of the substrate (7). Other work involved low-cost SERS substrate creation on a variety of materials from paper to silicon (3,4,8), the creation of inexpensive 3D-printed tools to improve the reliability of drop-casting in SERS (9) and testing various candidates for SERS substrate fabrication for their propensity to biofoul in marine environments (10). While much of our SERS work originated in materials science, the most recent project started with a SERS focus—exploring N-heterocyclic carbenes to tune the surface chemistry of gold SERS substrates—but then directed us to a materials science focus: the carbenes could be used as molecular etchants for gold (11).
You’re an author or co-author on more than 50 peer-reviewed publications and 5 issued patents. Briefly talk about your first paper, as well as other memorable ones you’re associated with.
My first publication was as an undergraduate working with Prof. Kathy Gough at Brock University and then the University of Manitoba (12). Kathy introduced me to my favorite theory—the quantum theory of atoms in molecules (QTAIM)—for unravelling the wonders of the molecular world, to Raman spectroscopy, to computational methods, and to rigorous research. I recall how exciting it was to simply be “doing cool science”. When Kathy told me that I would be acknowledged as a co-author, I remember feeling a flood of excitement, pride, and accomplishment. It felt like a defining moment, a culmination of years of study and hard work, knowing that I had contributed to the advancement of knowledge. I remain so grateful for that opportunity. Kathy has remained a cherished mentor across all stages of my career and continues to inspire me with the work that she is doing.
I remember once reading an assertion that you could never experimentally determine a reaction mechanism. Although it was true in a restricted sense, the absolutism of that statement really bothered me. It stuck with me like a challenge. Years later I applied to graduate school and met Prof. Dwayne Miller (University of Toronto). He proposed a dream experiment of making a molecular movie camera capable of revealing the gymnastics of a chemical reaction in real time. If successful, this project would refute the very statement that had driven me since high school. Starting from a bag of bolts, we steadily built this camera over more than four years. It was a challenge with the setbacks and pernicious vagaries of working on the cutting-edge of science and engineering. It was also intellectually stimulating and formative—and it worked! Ultimately there was both relief and excitement when our first experimental result made the cover of Science in 2003 (13)!
My doctoral research was not entirely consumed by molecular moviemaking. I led a project championing nanofabrication as an enabling tool for ultrafast science. My colleagues were facing extreme difficulties in presenting the sample for the first multidimensional femtosecond infrared spectroscopy experiments on pure liquid water. I invented a nanofluidic sample cell that made it possible to do those already challenging experiments. Our publication in Nature (14) revealed, as one reviewer succinctly noted, that pure water acted nothing like the model system! This sample cell eventually found its way to being the flagship product of a company that I co-founded, Insight Nanofluidics, Inc., and for which I led the corporate R&D program for half a decade after starting my faculty position.
Our 2018 Nature Communications paper (15) is special for being my group’s first demonstration of nanopore single-molecule sensing of glycans, and our first published experimental nanopore sensing paper. We had no idea whether any of the molecules (including enzymatic digestion products of some of those molecules) could even be detected by the nanopore method. That we could successfully sense a range of biologically compelling molecules displaying an exciting diversity of molecular structures and physicochemical properties was both thrilling and encouraging.
Finally, our 2022 ACS Nano paper (16) is special for its unexpected weaving together of all my earlier, formative research experiences, and for demonstrating the enabling nature of research collaboration. After inventing a new method to decorate the inner surface of our pores (17) (winner of the 2019 SciX Innovation Award), we were pursuing higher-performance coatings. I’ve long been enamored of photochromic molecules, especially given my “molecular movie camera” days where they prove to be the ideal, unflagging, multi-take performers. With a photochromic coating, we could get two nanopore coatings for the price of one, simply by using light to switch between the two different film configurations. Prof. Grace Han’s group at Brandeis University supplied the custom photochromic molecule and insight into the structural basis of its performance—insights that evoked memories of my undergraduate computational chemistry research. Understanding the microscopic details of how photoisomerization of the surface coating then switched the nanopore transport properties came about through lively discussions infused with reflections on my earlier work using femtosecond infrared spectroscopy to understand biopolymer hydration dynamics (Prof. Dr. Thomas Elsaesser & Dr. Erik Nibbering, Max Born Institute) and water structure (Prof. Dwayne Miller). We applied these molecularly-tuned nanopores to sense both DNA and glycans in ways that have downstream applications in biomedical sensing and molecular data storage even as the immediate observations could be used to further characterize how the coating configuration affected the fundamental nature of biopolymer transport through the nanopore. Far from being just an opportunity to reminisce about my journey through research and to enjoy the synergy of collaboration, this work also provides a firm foundation for continuing our work in using chemistry to improve nanopore single-molecule sensing.
According to your LinkedIn profile (18), your career has taken you from Canada to Germany, back to Canada, then to the United States. Any interesting “culture shock” stories you’d like to share? How have these travel experiences impacted you professionally and personally?
Living abroad in Germany was a transformative experience for me. The directness of communication was a balm. The routine of going for a relaxing lunch with colleagues was a wonderful part of daily working life. Combining this genial approach with science at the highest level was a heartening experience for me. I had colleagues and friends who helped me get set up for life in Germany. Even so, there were challenges that have given me a much greater appreciation for what many of our graduate students from abroad go through in pursuit of their own scientific dreams.
The most startling culture shock was that it was easier setting up my banking and immigration status in Germany than in the country I’d grown up 20 minutes across the border from. The most comical culture shock moment was forgetting to switch from “Thanksgiving” and “American Thanksgiving” to “Canadian Thanksgiving” and “Thanksgiving”. Having already celebrated the Canadian holiday, I inadvertently tried to schedule a midterm on that sacrosanct American holiday! I can still see the abject looks of horror and stirrings of rebellion!
What are the pros and cons of your work in a laboratory environment, and your work in a classroom environment?
I’m an adherent of doing science in service to society. Both research and teaching are a part of that, and I try to pass this ethos of service on to the next generation. Having worked in both academia and the startup sector, I understand the challenges and opportunities of transferring discoveries from the laboratory into real-world solutions. I enjoy infusing my teaching and research with society-facing context. I try to bring the problem-solving we do to advance our research into the classroom, too, particularly in lab classes. It’s a fun part of the job to emphasize that science translation piece. It also helps to support my overarching mission to help students realize how science and effective science-based communication can help address a myriad of social challenges.
Long ago, my parents gave me a refrigerator magnet with the words “To teach is to touch a life forever.” Those words are both sobering and uplifting. What keeps me inspired is that it’s both the greatest gift and greatest responsibility to help students learn how to think, especially in ways that transcend conventional views of academia.
There are some challenges in both environments. Just as we all agonize in our research, there are times when that happens over lesson plans. There’s just so much exciting science and application to share and never enough classroom time to share it! The laboratory, both for research and teaching, can be one of the most exciting places to be because it gives you access to delve into the mysteries of the molecular world—if you pay proper attention to the fundamentals. It’s tough to design undergraduate experiments that appropriately combine a sense of rigor and exploration. In an ideal world, I’d let the students discover and explore first principles through play at the edges of their imaginations, without being constrained by the syllabus or course calendar.
What are some of your hobbies? What do you like doing when you’re not in the classroom or the laboratory?
I’m a lifelong practitioner, as both student and teacher, of the traditional Japanese martial arts judo and jiu-jitsu. It was a special moment when I visited Japan as an invited speaker and immersed myself in the culture that had been so formative for me since I was 7 years old. Closer to home, I have the good fortune to live minutes from the water and enjoy kayaking in it, or rollerblading alongside it. In colder weather, I’ll often take a midday break ice skating at the university rink. At rest, I’m an avid reader with eclectic tastes, but I always come back to reading works of history. I’m soon going to see if blacksmithing will make it onto my keeper list of hobbies.
What do you think (or hope) that your students and colleagues would say about you?
That I care deeply about, and am invested in, supporting them in their growth as scientists and, broadly, as people. That I’m committed to integrity, collegiality, and lifting people up. The thrill of discovery, publishing papers, and earning recognition are rewarding and meaningful, but I measure my impact in the lives that I touch. Seeing students grow and reach their goals resonates with me in a profound way. I’m still so thrilled that two of my PhD graduates, Nuwan Bandara and Buddini Karawdeniya, are on their way as independent faculty members in chemistry and biomedical engineering at Ohio State University.
If you never became a scientist, what other fields do you see yourself exploring?
I spent a long time being drawn to teaching high school science. I was fortunate to have been featured in a Discovery Channel documentary while in graduate school, and—perhaps channeling my early aspirations to host “Jeopardy”—science communication jobs remain an intriguing prospect. Ultimately my current position gives me the flexibility to incorporate science communication through research, teaching, and public outreach. As an undergraduate student, I spent some time considering pursuing a degree in French literature or linguistics. Starting in graduate school, I started to get really interested in the role that a Ph.D.-level scientist could play in the public policy space, whether the policy dealt explicitly with science or not. These days that has transitioned into a strong interest in technology transfer and improving the connections between universities and industry.
What does receiving the AES Mid-Career Award mean to you?
The AES Electrophoresis Society’s name doesn’t do justice to the scope of expertise spanning electrokinetics, separations, device physics, microfabrication, and microfluidics. I’d had no formal training in those fields when one of their session titles at SciX caught my eye as being a perfect match to our work. At that first meeting, I was thrilled to find an engaging community which opened me up to a wide range of scientific and engineering possibilities, discoveries and applications—not to mention such a tremendous amount of expertise! Reflecting on that history with AES, it is extraordinarily gratifying to have gone from being welcomed to being awarded by the society. My group has been working for well over a decade doing discovery and development work in nanofluidics, materials science, single-molecule sensing, and of course electrokinetics, device physics and more. We’ve been working to expand and improve the suite of tools available for sensing glycans, especially, alongside other biologically important molecule classes. It’s meaningful to receive this recognition, which I share with my group, of the quality and significance of our work from an organization that leads and stewards a field of such impressive diversity and scope. It is wonderful to be awarded this opportunity to highlight the noteworthy science and engineering progress of my group. On a personal level, it has been rewarding to reflect on past projects and savor that while my work is purpose-driven, I’ve also had a lot of fun doing some really cool science!
As this is a mid-career award, where do you anticipate/hope your career will take you?
When I got tenure, I was an experimental nanopore scientist who hadn’t yet published an experimental nanopore sensing paper. Our first manuscript on the subject (15) was, I hope, an audacious experimental demonstration of what nanopore single-molecule sensing could do for the analysis of glycans (sugars). We surveyed several different glycans including their enzymatic digestion products to explore what types of glycans could be analyzed and how molecular composition, structure, and properties affected the sensing performance. We also successfully tackled a pharmaceutical quality assurance problem: detecting an adulterant in the ubiquitous clinical therapeutic heparin—a toxin which had eluded detection until around 100 people in the U.S. died in 2008. This early work demonstrated the need to be able to chemically tune nanopore surfaces, and we invented a simple, nearly bulletproof way of doing this not long after (17). In follow-on work, we worked with the Robert Linhardt group and Rensselaer Polytechnic Institute and the Grace Han group at Brandeis to demonstrate single-monomer-resolved nanopore glycan characterizations (19)—the first step needed for glycan sequencing—and the installation and use of a photoresponsive nanopore surface coating, respectively (16). We are now working to unite our ability to chemically decorate nanopores with our need to have finely controlled surface interactions with the glycans passing through our nanopores. In the longer term, we are pushing to expand the analyte scope beyond glycans and beyond even glycoproteins: we are seeking to be able to design and fabricate “smart” nanopores to support the much wider application of nanopore single molecule sensing (20,21). My long-standing interest in the richness of molecular structure and the elegance and beauty of molecular function and interactions remains central and will guide this ongoing journey of exploration and discovery.
Jason R. Dwyer is a Professor of Chemistry at the University of Rhode Island focusing on bioanalytical chemistry, nanofabrication, and technology development for the life sciences. Trained as a physical chemist (Ph.D. University of Toronto, 2005), he has worked as a biophysicist (NSERC postdoctoral fellow at the Max Born Institute, Berlin, Germany), applied biophysicist (University of British Columbia), nanoscientist, scientific entrepreneur, and consultant in the life sciences and instrumentation sectors. His current research spans those interests and targets both discovery and application. A principal focus of his research is to advance nanopores from a thin-film nanofluidic sensor platform primarily used for sequencing DNA to a single-molecule-sensitive enabling tool for glycomics—the study of the structure and function of complex sugars. Professor Dwyer’s work at the intersection of nanopore glycomics and molecular-level tuning of nanopore function was recognized with the 2019 FACSS Innovation Award at SciX. Materials science discoveries arising from this primary research effort underpin a second research thrust in technology development for surface-enhanced Raman spectroscopy (SERS). He has been recognized for his commitment to intentional, value-driven mentoring that strives for uplift both individually and broadly. Alongside more than 50 publications, 5 issued patents, and over 80 invited lectures, he co-founded and directed a nanotechnology start-up company, Insight Nanofluidics, and engaged in outreach activities with multiple media outlets including a feature documentary with the Discovery Channel. At its heart, Professor Dwyer’s research group is interested in learning about molecules, how they’re put together, how they work, and how they interact with each other—and applying that knowledge to benefit society. Photo courtesy of Michael Salerno.
References
1. Division of Research and Economic Development. University of Rhode Island webpage. https://web.uri.edu/research-admin/jason-dwyer/ (accessed 2024-05-22)
2. Dwyer Research Group. University of Rhode Island webpage.
https://dwyergroup.wixsite.com/dwyerresearchgroup (accessed 2024-05-22)
3. Whelan, J. C.; Karawdeniya, B. I.; Bandara, Y. M. N. D. Y.; Velleco, B. D.; Masterson, C. M.; Dwyer, J. R. Electroless Plating of Thin Gold Films Directly onto Silicon Nitride Thin Films and into Micropores. ACS Appl. Mater. Interfaces 2014, 6 (14), 10952-10957. DOI: 10.1021/am501971n
4. Sheetz, B. S.; Bandara, Y. M. N. D. Y.; Rickson, B.; Auten, M.; Dwyer, J. R. Rapid, General-Purpose Patterning of Silicon Nitride Thin Films Under Ambient Conditions for Applications Including Fluid Channel and SERS Substrate Formation. ACS Appl. Nano Mater. 2020, 3 (3), 2969-2977. DOI: 10.1021/acsanm.0c00248
5. Bandara, Y. M. N. D. Y.; Karawdeniya, B. I.; Dwyer, J. R. Push-Button Method To Create Nanopores Using a Tesla-Coil Lighter. ACS Omega 2019, 4 (1), 226-230. DOI: 10.1021/acsomega.8b02660
6. Chevalier, R. B.; Dwyer, J. R. An Open Source, Iterative Dual-Tree Wavelet Background Subtraction Method Extended from Automated Diffraction Pattern Analysis to Optical Spectroscopy. Appl. Spectrosc. 2019, 73 (12), 1370-1379. DOI: 10.1177/0003702819871330
7. Chevalier, R. B.; Dwyer, J. R. Optimizing Noncontact Oxygen-Plasma Treatment to Improve the Performance of a Top-Down Nanofabricated Surface Enhanced Raman Spectroscopy Substrate with Structurally rResponsive, High-Aspect-Ratio Nanopillar Array. J. Raman Spectrosc. 2021, 52 (3), 608-615. DOI: https://doi.org/10.1002/jrs.6050
8. Karawdeniya, B. I.; Bandara, Y. M. N. D. Y.; Whelan, J. C.; Dwyer, J. R. General Strategy To Make an On-Demand Library of Structurally and Functionally Diverse SERS Substrates. ACS ACS Appl. Nano Mater. 2018, 1 (2), 960-968. DOI: 10.1021/acsanm.7b00385
9. Karawdeniya, B. I.; Chevalier, R. B.; Bandara, Y. M. N. D. Y.; Dwyer, J. R. Targeting Improved Reproducibility in Surface-Enhanced Raman Spectroscopy with Planar Substrates Using 3D Printed Alignment Holders. Rev. Sci. Instrum. 2021, 92 (4), 043102. DOI: 10.1063/5.0039946
10. Kurtz, K. R.; Thalyta Silva de Oliveira, T.; Chevalier, R.; Rayes, N.; Bose, A.; Dwyer, J. R.; Oyanedel-Craver, V. Biofouling Potential of Surface-Enhanced Raman Scattering-Based Seawater Quality Sensors by Ulva spp. Biofouling 2023, 39 (6), 629-642. DOI: 10.1080/08927014.2023.2242283
11. Chevalier, R. B.; Pantano, J.; Kiesewetter, M. K.; Dwyer, J. R. N-Heterocyclic Carbene-Based Gold Etchants. Beilstein J. Nanotechnol. 2023, 14, 865–871. DOI: 10.3762/bjnano.14.71
12. Gough, K. M.; Yacowar, M.; Cleve, R. H.; Dwyer, J. R. Analysis of Polarizability Derivatives in H2, HF, F2, N2 and CO with the Theory of Atoms in Molecules. Can. J. Chem. 1996, 74, 1139-1144. DOI:10.1139/v96-128
13. Siwick, B. J.; Dwyer, J. R.; Jordan, R. E.; Miller, R. J. D. An Atomic-Level View of Melting Using Femtosecond Electron Diffraction. Science 2003, 302 (5649), 1382-1385. DOI: 10.1126/science.1090052
14. Cowan, M. L.; Bruner, B. D.; Huse, N.; Dwyer, J. R.; Chugh, B.; Nibbering, E. T. J.; Elsaesser, T.; Miller, R. J. D. Ultrafast Memory Loss and Energy Redistribution in the Hydrogen Bond Network of Liquid H2O. Nature 2005, 434 (7030), 199-202. DOI: 10.1038/nature03383
15. Karawdeniya, B. I.; Bandara, Y. M. N. D. Y.; Nichols, J. W. et al. Surveying Silicon Nitride Nanopores for Glycomics and Heparin Quality Assurance. Nat. Commun. 2018, 9, 3278. https://doi.org/10.1038/s41467-018-05751-y
16. Hagan, J. T.; Gonzalez, A.; Shi, Y.; Han, G. G. D.; Dwyer, J. R. Photoswitchable Binary Nanopore Conductance and Selective Electronic Detection of Single Biomolecules Under Wavelength and Voltage Polarity Control. ACS Nano 2022, 16 (4), 5537-5544. DOI: 10.1021/acsnano.1c10039
17. Bandara, Y. M. N. D. Y.; Karawdeniya, B. I.; Hagan, J. T.; Chevalier, R. B.; Dwyer, J. R. Chemically Functionalizing Controlled Dielectric Breakdown Silicon Nitride Nanopores by Direct Photohydrosilylation. ACS Appl. Mater. Interfaces 2019, 11 (33), 30411-30420. DOI: 10.1021/acsami.9b08004
18. Jason Dwyer LinkedIn page. https://www.linkedin.com/in/jason-dwyer-31029538/ (accessed 2024-05-22)
19. Xia, K.; Hagan, J. T.; Fu, L.; Sheetz, B. S.; Bhattacharya, S.; Zhang, F.; Dwyer, J. R.; Linhardt, R. J. Synthetic Heparan Sulfate Standards and Machine Learning Facilitate the Development of Solid-State Nanopore Analysis. Proc. Natl. Acad. Sci. U. S. A. 2021, 118 (11), e2022806118. DOI: 10.1073/pnas.2022806118
20. Kizer, M. E.; R. Dwyer, J. Editors’ Choice—Perspective—Deciphering the Glycan Kryptos by Solid-State Nanopore Single-Molecule Sensing: A Call for Integrated Advancements Across Glyco- and Nanopore Science. ECS Sensors Plus 2024, 3 (2), 020604. DOI: 10.1149/2754-2726/ad49b0
21. Hagan, J. T.; Sheetz, B. S.; Bandara, Y. M. N. D. Y.; Karawdeniya, B. I.; Morris, M. A.; Chevalier, R. B.; Dwyer, J. R. Chemically Tailoring Nanopores for Single-Molecule Sensing and Glycomics. Anal. Bioanal. Chem. 2020, 412 (25), 6639-6654. DOI: 10.1007/s00216-020-02717-2