Researchers Uncover Crucial Immune Defense Mechanism in Bacteria
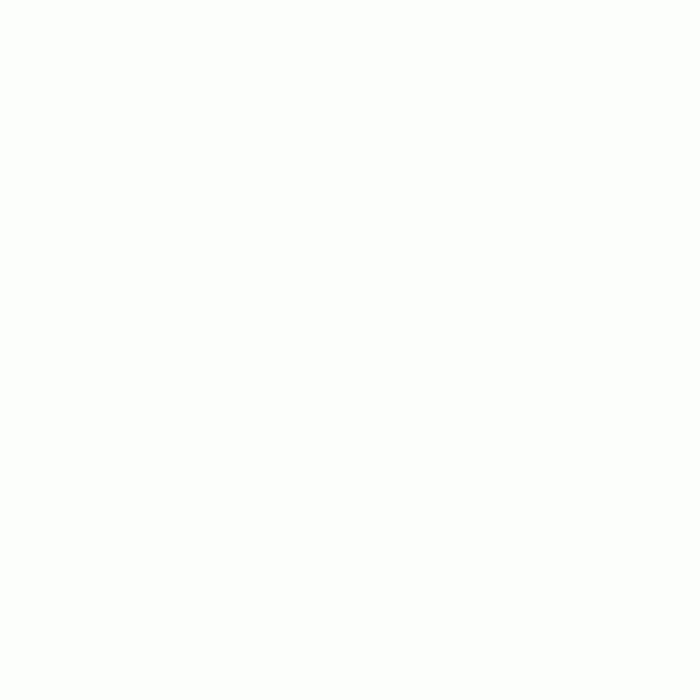
In a groundbreaking leap for microbiology and immunology, a collaborative research team from the Institute of Biophysics of the Chinese Academy of Sciences and the Beijing Institute of Technology has unveiled a sophisticated molecular mechanism that elucidates how bacteria mount a robust defense against viral invaders. Published recently in the prestigious journal Cell, this study sheds light on the elusive processes that govern bacterial immunity via the cyclic oligonucleotide-based anti-phage signaling system, or CBASS, offering unprecedented insights into innate antiviral responses at a molecular level.
At the heart of this discovery lies the role of cyclic dinucleotides (CDNs), small signaling molecules synthesized by bacteria in response to phage infection. These CDNs act as molecular alarms that trigger the bacterial immune system, yet until now, the detailed pathways connecting CDN sensing to immune effector activation remained largely uncharted. The research team has revealed that CDNs initiate a transformative process that culminates in the assembly of filamentous structures composed of phospholipase effectors, particularly focusing on CapE, a key phospholipase integral to CBASS function.
Employing a sophisticated integrative methodology combining cryo-electron microscopy with X-ray crystallographic analysis, scientists were able to capture CapE in three distinct conformational states. The inactive dimer form represents the quiescent baseline, while the intermediate CDN-bound state unveils a complex higher-order assembly indicative of activation. Finally, the substrate-analog-bound catalytic mimic state illustrates the enzyme primed for its biochemical role. These structural snapshots not only clarify the dynamic shifts in CapE but also serve as a rare glimpse into the stepwise activation mechanics within bacterial immune pathways.
Upon binding CDNs, CapE undergoes a dramatic conformational rearrangement that reveals its previously occluded catalytic site. This exposure is pivotal for enzymatic activity, as it enables CapE molecules to polymerize into filamentous assemblies. These filaments constitute active platforms strategically oriented to assault bacterial membranes by hydrolyzing phospholipids. This membrane disruption acts as a decisive defense, incapacitating phage propagation through self-induced programmed cell death, thereby protecting the bacterial population at large.
What distinguishes this system is the filamentous assembly itself — a structural motif that amplifies enzymatic function and enforces spatial organization necessary for effective membrane targeting. This phenomenon of effector filamentation as a regulatory mechanism resonates beyond bacterial immunity and is increasingly recognized as a recurring theme in innate immune systems across the evolutionary spectrum. The study posits that such filament formation serves not merely as a bacterial adaptation but as a broadly conserved strategy for enzymatic regulation.
To validate the functional relevance of these findings, the team conducted structure-guided mutagenesis experiments. Targeted mutations that impaired filament formation or enzymatic capability resulted in significantly diminished bacterial resistance, underscoring the indispensable role of both polymerization and catalytic activity in CBASS-driven immunity. Such experimental confirmation cements the model linking CDN signaling to effector activation and membrane-targeted immune responses as integral to bacterial survival strategies.
The implications of this research extend far beyond basic biology. Understanding how bacteria sense and respond to viral threats at a molecular scale offers avenues for novel antimicrobial strategies, particularly in an era where antibiotic resistance poses a growing threat. By manipulating or mimicking these immune pathways, we might develop innovative therapies that bolster beneficial bacteria or disrupt harmful pathogens. Furthermore, the mechanisms unraveled by this study may inspire biomimetic approaches in synthetic biology and nanotechnology, harnessing filamentous protein assemblies for tailored molecular functions.
Crucially, this work bridges a critical knowledge gap that has persisted in the field of prokaryotic immunity. While CBASS had been recognized as vital to antiviral defense, the molecular choreography linking CDN sensing to phospholipase activation was speculative at best. This research offers a unified conceptual framework, integrating ligand sensing, protein polymerization, and enzymatic disruption in a seamless narrative that accounts for the rapid and potent immune responses observed in bacteria.
The structural elucidation of CapE’s conformational dynamics offers compelling evidence of how bacterial enzymes leverage multimeric assemblies to regulate activity and specificity. These insights rekindle interest in exploring protein filamentation as a regulatory paradigm not only in bacteria but also in more complex eukaryotic immune processes, implying an evolutionary conservation that may inform a broad swathe of immunological research.
Moreover, the study enriches our understanding of programmed cell death in prokaryotes, a phenomenon increasingly recognized as a sophisticated form of altruism at the cellular level. By triggering membrane disruption through CapE’s phospholipase activity, infected bacterial cells effectively sacrifice themselves to prevent viral dissemination, showcasing the intricate balance bacteria maintain between individual survival and population-level immunity.
This compelling narrative of bacterial self-defense broadens our comprehension of immunity as a universal biological imperative, demonstrating that even the simplest organisms have evolved intricate strategies to detect and repel viral threats. It further exemplifies how modern structural biology can elucidate the fine molecular details that underpin complex biological systems, translating microscopic events into macroscopic understanding.
In conclusion, the research led by GAO Pu and colleagues represents a seminal contribution to the field of innate immunity, revealing the molecular basis of a critical antiviral defense mechanism in bacteria. Through meticulous structural and functional analyses, it demonstrates the centrality of cyclic dinucleotide-induced phospholipase polymerization in activating membrane-targeting immune responses. This discovery paves the way for new exploratory avenues in microbiology, immunology, and biotechnology, highlighting the enduring power of nature’s molecular architectures.
Subject of Research: Cells
Article Title: Cyclic dinucleotide-induced filamentous assembly of phospholipases governs broad CBASS immunity
News Publication Date: 8-May-2025
Web References: https://doi.org/10.1016/j.cell.2025.04.022
Image Credits: GAO Pu’s group
Keywords: Cell biology, Immunology, Cellular physiology, Immune cells
Tags: antiviral responses in microbiologybacterial defense against viral invadersbacterial immune defense mechanismsCapE phospholipase functionCBASS signaling system in bacteriacollaborative research in microbiologycryo-electron microscopy in microbiologycyclic dinucleotides in bacteriainnate antiviral mechanismsmolecular pathways in bacterial immunityphage infection and bacterial immunityX-ray crystallography in immunology