Molecular communication: Experts are interpreting the language of life
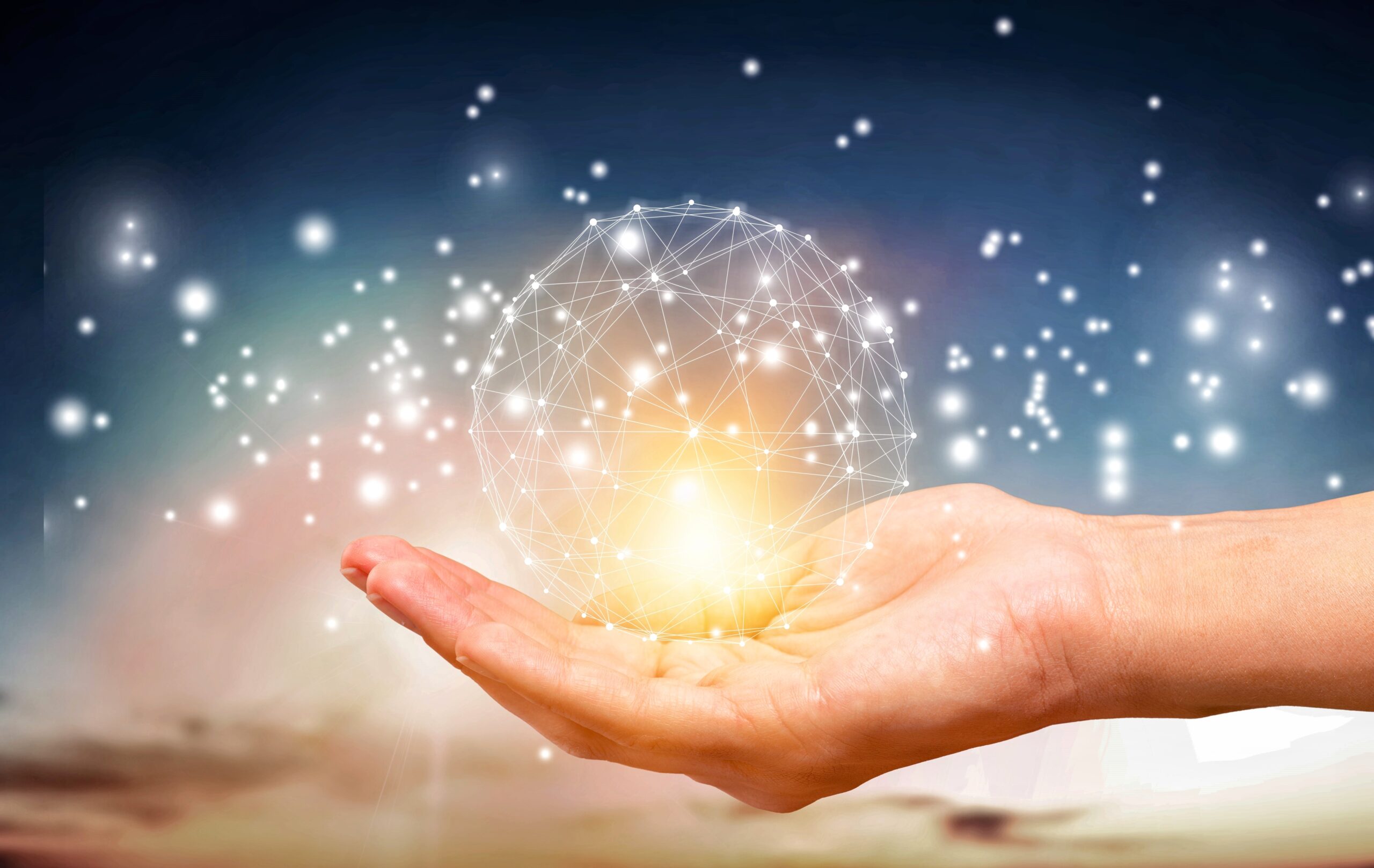
Canadian researchers at the Université de Montréal have managed to recreate and mathematically validate two crucial molecular languages believed to be at the very core of life itself. This new insight into molecular communication, recently published in the Journal of the American Chemical Society, is a monumental leap toward the future of nanotechnologies.
The research may ultimately expand the horizons of nanotechnology applications, from enhanced biosensing and drug delivery systems to innovative molecular imaging techniques.
Focus of the study
The complexity of life is rooted in the billions of nanomachines and nanostructures that constitute living organisms.
These minuscule entities engage in sophisticated forms of communication, culminating in the creation of higher-order entities capable of movement, cognition, reproduction, and survival.
Understanding molecular communication
“The key to life’s emergence relies on the development of molecular languages – also called signaling mechanisms – which ensure that all molecules in living organisms are working together to achieve specific tasks,” explained Professor Alexis Vallée-Bélisle, the lead investigator on the study.
In yeasts, for example, upon detecting and binding a mating pheromone, billions of molecules will communicate and coordinate their activities to initiate union, said Professor Vallée-Bélisle.
“As we enter the era of nanotechnology, many scientists believe that the key to designing and programming more complex and useful artificial nanosystems relies on our ability to understand and better employ molecular languages developed by living organisms.”
Elusive molecular languages
Two molecular languages have come into focus. The first, allostery, operates on the “lock-and-key” principle: a molecule attaches to and changes the structure of another, directing its actions.
The second language, known as multivalency or the chelate effect, is likened to puzzle pieces fitting together. The binding of one molecule to another makes it easier (or not) for a third molecule to attach by increasing its binding interface.
However, until now, a comprehensive understanding of the rules and intricacies of these languages remained elusive. “Given the complexity of natural nanosystems, before now nobody was able to compare the basic rules, advantages or limitations of these two languages on the same system,” said Professor Vallée-Bélisle.
Simple mathematical equations
To investigate, doctoral student Dominic Lauzon embarked on an innovative approach, using DNA as the basis for a molecular system capable of utilizing both languages. He said that DNA is like Lego bricks for nanoengineers. “It’s a remarkable molecule that offers simple, programmable and easy-to-use chemistry.”
The study revealed that both of these languages could be distilled down to simple mathematical equations. This unraveled the principles needed to facilitate communication between molecules within nanosystems.
As the team delved deeper, they tapped into the language of multivalency to design a state-of-the-art antibody sensor, capable of detecting varying concentrations of antibodies.
“As shown with the recent pandemic, our ability to precisely monitor the concentration of antibodies in the general population is a powerful tool to determine the people’s individual and collective immunity,” noted Professor Vallée-Bélisle.
More about molecular communication
Molecular communication is an incredibly intricate process that is central to the functioning of all living organisms. It involves the transmission, reception, and processing of information between molecules to facilitate coordinated biological processes.
Nature’s mode of communication
Molecular communication is the primary means through which cells and organisms communicate. For instance, neurotransmitters facilitate communication between nerve cells, while hormones serve as messengers carrying information throughout the body.
Molecular signals
Molecular signals can take the form of small molecules, proteins, ions, or even changes in electrical potential. These signals are often specifically tailored to fit receptor molecules like a key fits a lock, ensuring precision in communication.
Chemical synapses
One of the most well-known examples of molecular communication is in chemical synapses in the nervous system. Neurotransmitters are released from the end of one neuron and travel across the synaptic gap to bind with receptors on another neuron. This binding can result in the transmission of a nerve impulse.
Hormonal signaling
Endocrine cells release hormones into the bloodstream. These hormones travel throughout the body, binding to specific cells and eliciting specific responses. For instance, insulin is a hormone that regulates glucose uptake by cells.
Autocrine and paracrine signaling
In autocrine signaling, cells produce signals that they themselves respond to. In paracrine signaling, cells produce signals that affect nearby cells. An example is the release of growth factors that stimulate cell growth or division in neighboring cells.
Quorum sensing in bacteria
Bacteria can “communicate” using a method called quorum sensing. When bacterial cells reach a certain concentration, they produce and release signaling molecules. Once these molecules reach a threshold concentration, the bacteria undergo a coordinated response, such as forming a biofilm.
Importance in medicine and biotechnology
Understanding molecular communication pathways can provide insights into many diseases, including cancer, where signaling pathways can become altered. In biotechnology, scientists can manipulate these pathways to produce desired outcomes, such as bacteria engineered to produce human insulin.
Nanotechnology and synthetic biology
Molecular communication is increasingly becoming crucial in fields like nanotechnology and synthetic biology. Researchers are exploring ways to use natural molecular communication systems to design and program artificial nanosystems. This has potential applications in targeted drug delivery, environmental monitoring, and even data storage.
Challenges
There’s still much to learn about molecular communication. The complexity of biological systems means that even small changes in one pathway can have cascading effects throughout an organism.
—-
Check us out on EarthSnap, a free app brought to you by Eric Ralls and Earth.com.